Unraveling the Intricacies of Electron Localization in Atomic Structures
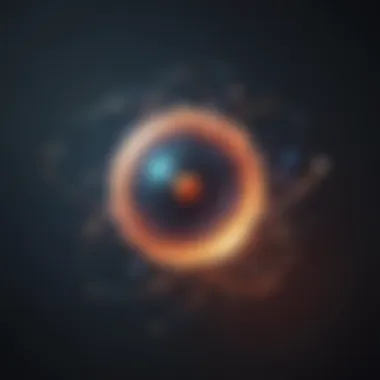
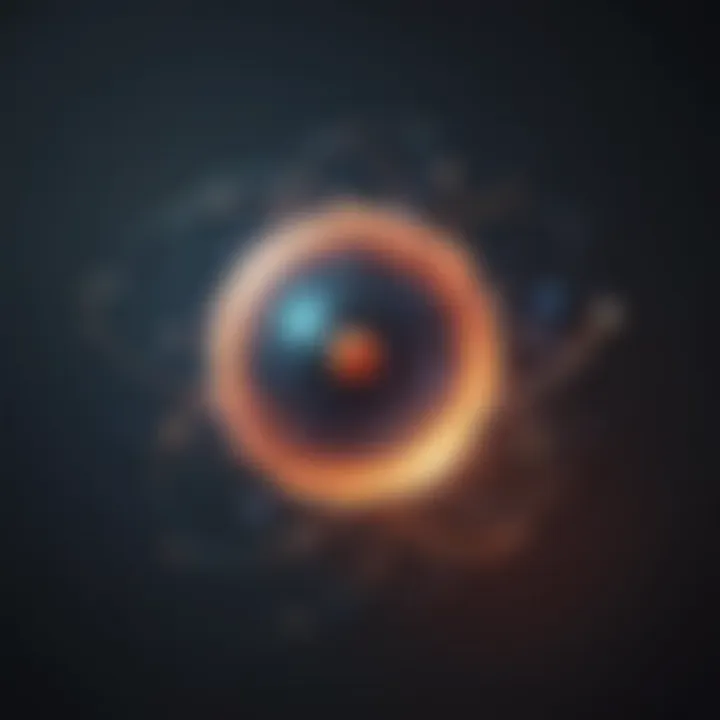
Technology Insights
In delving deep into the intricacies of electron location within atomic structures, we find ourselves at the intersection of cutting-edge technology and foundational scientific principles. The latest tech trends have significantly influenced the way we study and visualize electron behavior, providing innovative tools and methods that enable us to explore the microscopic world with unprecedented detail and precision.
Innovation in Tech
One of the most groundbreaking innovations in electron location research is the development of advanced microscopy techniques, such as high-resolution transmission electron microscopy, scanning tunneling microscopy, and atomic force microscopy. These cutting-edge technologies have revolutionized our understanding of electron distribution within atoms, offering researchers a closer look at the spatial arrangement of electrons in various atomic structures.
Entertainment Highlights
As we uncover the mysteries of electron location, we embark on a captivating journey through the realms of atomic and molecular behavior. While this subject may seem far removed from the glitz and glamour of the entertainment industry, the parallels between unveiling electron locations and celebrity news are surprisingly relevant.
Movie Reviews
Just as movie reviews dissect the nuances of cinematic performances, electron location studies deconstruct the intricate roles electrons play in chemical reactions and molecular dynamics. Each electron position is akin to a character in a film, contributing uniquely to the overall plot and outcome of chemical processes.
Design Showcase
Exploring electron location is akin to unraveling the creative designs found in the building blocks of matter. Atomic and molecular structures exhibit architectural trends that rival the most avant-garde designs in the world of art and architecture.
Architectural Trends
The spatial arrangements of electrons within atoms mirror the innovative architectural trends shaping our cities and structures. Just as architects push the boundaries of design with bold and unconventional structures, electrons challenge our perception of spatial distribution with their nuanced positioning within atomic orbits.
Industry Spotlights
In the quest to uncover the intricate details of electron location, interviews with tech experts shed light on the groundbreaking discoveries and developments in the field of atomic and molecular research. These behind-the-scenes glimpses into the minds of designers and innovators provide valuable insights into the inner workings of electron behavior.
Behind-the-Scenes in Entertainment
While electron location may seem like a distant concept to those ensconced in the world of entertainment, the parallels between the meticulous craftsmanship of designers and the precise positioning of electrons are striking. Just as entertainment shines a spotlight on creativity and talent, the study of electron location highlights the ingenuity and complexity of the microscopic world.
Event Coverage
Tech conferences and design exhibitions serve as platforms for showcasing the latest advancements and innovations in electron location research. These events provide a comprehensive overview of the cutting-edge technologies and methodologies driving the exploration of electron behavior within atomic structures.
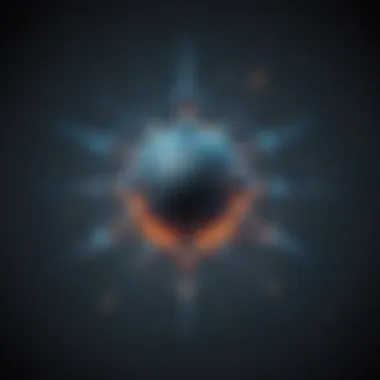
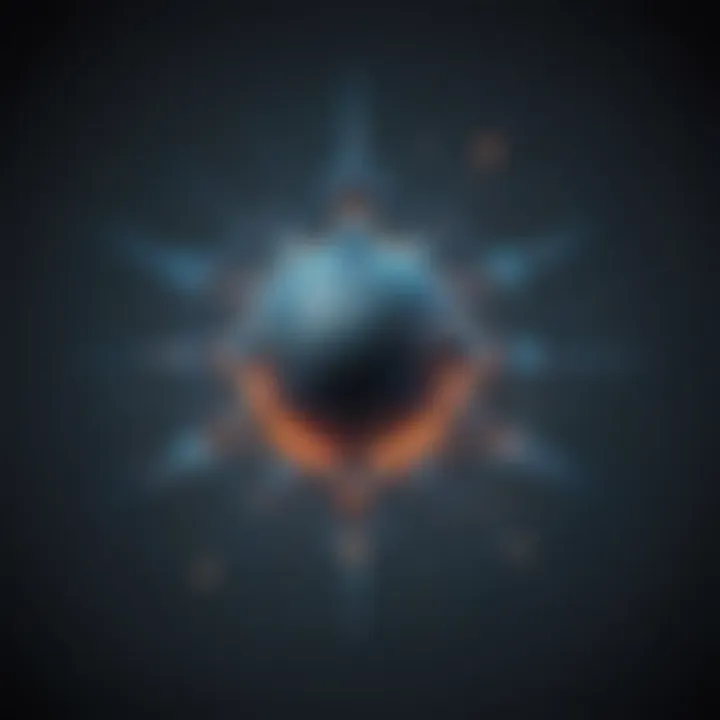
Design Exhibitions Highlights
Much like the dazzling displays at design exhibitions, electron location studies unveil the beauty and complexity inherent in the microscopic realm. Attendees of these exhibitions witness firsthand the intricate patterns and structures that govern electron positions within atoms, offering a window into the mesmerizing world of quantum mechanics and atomic interactions.
Electron location is a fundamental concept in the realm of atomic and molecular sciences, shedding light on the precise positioning of electrons within different atomic structures. This article unveils the mysteries surrounding electron behavior in atoms and molecules, offering key insights into various chemical and physical processes.
Fundamentals of Electron Location
The Concept of Electrons
Electrons, the negatively charged subatomic particles, play a pivotal role in determining the chemical and physical properties of elements. The concept of electrons revolves around their ability to occupy specific energy levels around the atomic nucleus, influencing bonding patterns and reactivity. Understanding the behavior of electrons is crucial in deciphering the complexities of atomic structures and chemical interactions. Although abstract, the concept of electrons serves as the foundation for comprehending electron location within atoms and molecules.
Importance of Electron Location
The importance of electron location lies in its implications for molecular stability, reactivity, and structure. By pinpointing the exact positions of electrons within an atom, scientists can predict how atoms will bond and interact with each other. Electron location is central to understanding electron configuration, which in turn governs an element's chemical behavior. Without a clear grasp of electron location, it would be challenging to grasp the intricacies of chemical reactions and material properties.
Atomic Structure
Electron Orbitals
Electron orbitals refer to the regions around an atomic nucleus where electrons are likely to be found. These three-dimensional spaces come in various shapes and sizes, each representing a different energy level for electrons. Understanding electron orbitals provides valuable insights into how electrons are arranged within atoms, influencing their chemical behavior and reactivity. The concept of electron orbitals forms the basis of theories like the Aufbau principle and Hund's rule, guiding our understanding of atomic structure and bonding.
Electron Cloud Model
The electron cloud model represents a modern approach to visualizing electron distribution within atoms. Unlike the traditional Bohr model, which depicts electrons orbiting the nucleus in fixed paths, the electron cloud model portrays electrons as existing within a diffuse cloud of probability. This model accounts for the wave-like behavior of electrons, offering a more accurate representation of their positions and energies within atomic structures. By embracing the electron cloud model, scientists can better comprehend the fuzzy nature of electron location and its impact on chemical bonding and reactivity.
Electron Localization Functions
In the realm of atomic structures lies the critical concept of Electron Localization Functions. Within the intricate world of electrons, understanding their localization functions is paramount. This section delves deep into the significance of Electron Localization Functions in shedding light on the behavior of atoms and molecules in various chemical and physical processes. By focusing on specific elements such as electron density and quantum mechanics, we unravel the complexities that govern electron behavior. Exploring Electron Localization Functions provides a nuanced understanding of electron distribution and its implications on atomic and molecular interactions.
Electron Density
Electron Probability Density
Electron Probability Density, a fundamental aspect of electron density, plays a pivotal role in our exploration of electron location. This specific element contributes significantly to our overall understanding of electron localization functions by providing insights into the likelihood of an electron's presence in a given region. The key characteristic of Electron Probability Density lies in its ability to predict the probability of finding an electron at a specific point within an atomic structure. This predictive capability proves immensely beneficial in interpreting electron behavior and its influence on chemical and physical phenomena. Despite its advantages, Electron Probability Density also has its limitations, particularly in accurately pinpointing electron locations in highly dynamic systems, a challenge that researchers continue to grapple with in this field of study.
Electric Charge Distribution
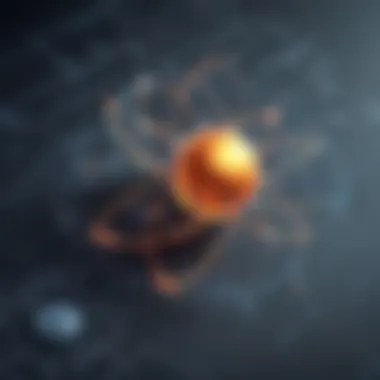
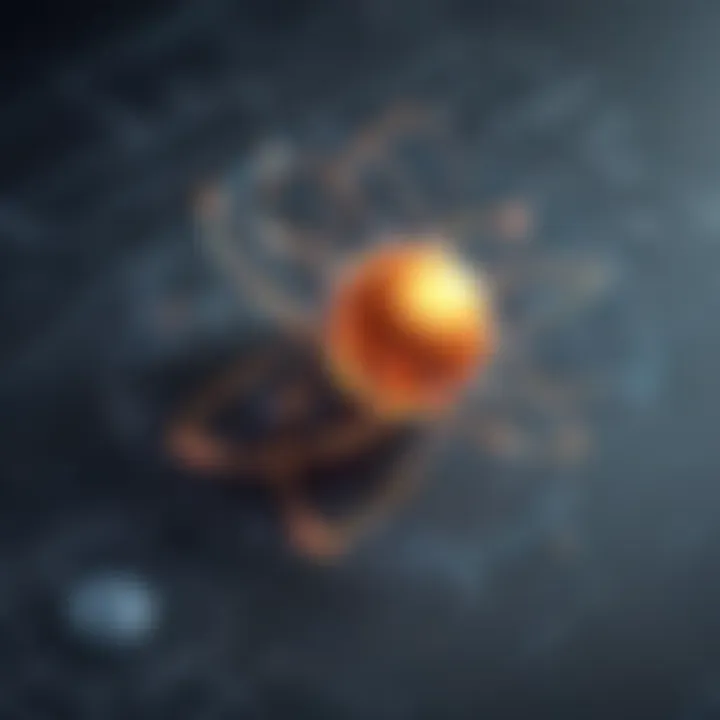
Another vital component of electron density is the Electric Charge Distribution. This aspect further enriches our understanding of electron localization functions by highlighting the distribution of electric charge within atomic structures. The key characteristic of Electric Charge Distribution lies in its ability to showcase how electric charge is dispersed around atomic nuclei, giving us valuable insights into electron positioning and interactions. By examining this unique feature, we can better grasp the electrostatic forces at play within atoms and molecules, ultimately deepening our comprehension of electron behavior. However, like Electron Probability Density, Electric Charge Distribution also faces challenges in accurately representing charge distributions in complex molecular environments, necessitating advanced computational models for more precise calculations.
Quantum Mechanics
Wave Function
Quantum Mechanics introduces us to the concept of the Wave Function, a central aspect of understanding electron location and behavior. The Wave Function serves as a mathematical expression that describes the quantum state of a system, including electrons within atomic structures. Its key characteristic lies in its ability to provide insights into the probabilistic nature of electron localization, offering valuable predictions about electron positions and energies. This feature makes the Wave Function a popular choice in our exploration of electron location, as it allows us to unravel the quantum phenomena governing electron distribution. While the Wave Function brings great advantages in modeling electron behavior, it also poses challenges in representing complex systems accurately, requiring sophisticated computational methods for precise calculations.
Heisenberg Uncertainty Principle
The Heisenberg Uncertainty Principle stands as a fundamental concept in Quantum Mechanics, significantly contributing to our understanding of electron location and behavior. This principle highlights the inherent limitations in simultaneously measuring the position and momentum of a particle with absolute precision. Its key characteristic lies in revealing the inherent uncertainty in predicting both the position and momentum of electrons within atomic structures, emphasizing the probabilistic nature of quantum mechanics. While the Heisenberg Uncertainty Principle enhances our comprehension of electron behavior, its limitation in providing precise simultaneous measurements poses challenges in fully determining electron locations and trajectories. Overcoming these limitations requires innovative approaches and a deep understanding of quantum theory to navigate the uncertainties inherent in representing electron behavior within atomic structures.
Factors Influencing Electron Location
In this section, we delve into the crucial factors that influence electron location within atomic structures. Understanding these factors is paramount in grasping the intricate behavior of electrons in atoms and molecules. Factors like electron affinity, chemical environment, magnetic fields, temperature, and pressure play a significant role in determining electron location.
Electron affinity, as a focus of this article, holds immense importance in delineating how electrons interact with their surrounding environment. The chemical environment, constituted of various elements and compounds, exerts a profound influence on electron behavior. Its unique characteristics dictate electron distribution and movement, thereby impacting the overall electron location within molecular structures. The chemical environment serves as a crucial element in the study of electron location due to its ability to modulate electron interactions and reactions.
Moving on to magnetic fields, another prominent factor affecting electron location, we explore the distinctive role these fields play in electron manipulation. Their ability to alter electron paths and orientations underscores their significance in determining electron positions within atoms and molecules. Magnetic fields offer a unique advantage in studying electron location by providing a controlled environment for observing electron behavior.
Electron Affinity
Chemical Environment
Delving deeper into the role of the chemical environment, we analyze how the composition of a specific environment influences electron behavior. The chemical environment characterizes the surroundings in which electrons reside, affecting their distribution and mobility. Understanding the intricate interplay between electrons and the chemical environment is vital to unraveling electron location within atomic structures.
Magnetic Fields
Turning our attention to magnetic fields, we elucidate their impact on electron movement and arrangements. Magnetic fields exhibit a distinctive property of influencing the trajectory of electrons, thereby influencing their spatial localization within atoms and molecules. Exploring the intertwining dynamics of electrons and magnetic fields sheds light on the nuanced mechanisms underlying electron location.
Temperature and Pressure
Effect on Electron Motion
The fluctuations in temperature and pressure exert a profound effect on electron motion within atomic structures. Changes in these environmental factors directly influence the energy levels and vibrational patterns of electrons, thereby altering their location within atoms and molecules. The effect on electron motion signifies the dynamic nature of electron behavior under varying temperature and pressure conditions.
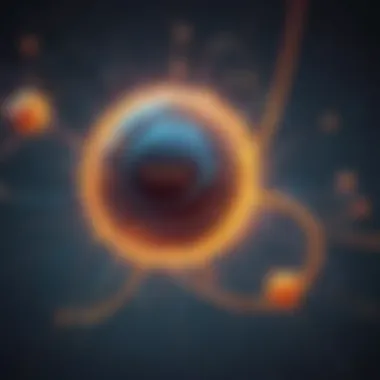
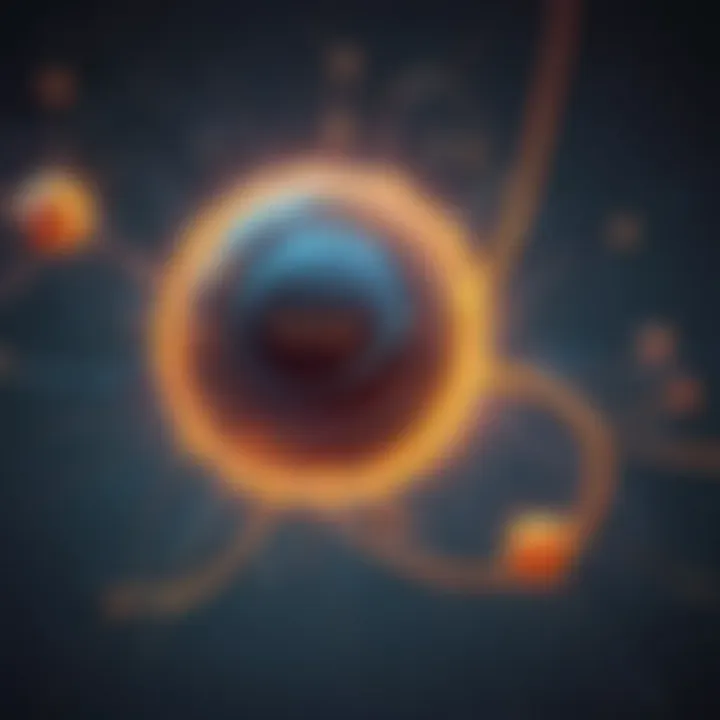
Phase Changes
Further, we examine how phase changes impact electron location within atomic structures. Transitions between different phases induce alterations in electron arrangements and interactions, leading to shifts in electron distribution. Analyzing the consequences of phase changes on electron location provides valuable insights into the role of physical states in determining electron positions.
Advanced Studies on Electron Location
The section on Advanced Studies on Electron Location delves deep into the importance of further exploration in this field within the broader context of electron location. Understanding the intricate details of electron behaviors and patterns within atomic structures is vital for unraveling the mysteries of chemical and physical processes. This section aims to shed light on the specialized techniques and methodologies that researchers employ to study electron location in depth and how these findings contribute to the advancement of scientific knowledge.
Spectroscopy Techniques
X-ray Photoelectron Spectroscopy
X-ray Photoelectron Spectroscopy (XPS) plays a pivotal role in the analysis of electron location within atomic structures. This technique provides valuable insights into the excitation and ejection of electrons from sample surfaces, offering researchers a powerful tool to investigate the chemical composition and electronic states of materials. The key characteristic of XPS lies in its ability to determine the energy levels of electrons, allowing for precise examination of electron interactions within complex molecular structures. Despite its limitations in probing deeper electron regions, XPS remains a popular choice in this article due to its high sensitivity and non-destructive nature. Its unique feature lies in the identification of elemental compositions and chemical states at a surface level, making it an indispensable tool for studying electron behavior in various materials.
Electron Energy Loss Spectroscopy
Electron Energy Loss Spectroscopy (EELS) offers a detailed perspective on electron interactions and excitations within atomic structures. By analyzing the energy loss of electrons during their passage through a specimen, EELS provides crucial information regarding electronic configurations and bonding characteristics. The key strength of EELS lies in its capability to map out the energy loss spectrum, enabling researchers to probe into the electronic structure and dynamics of materials with high precision. This technique is favored in this article for its ability to investigate localized electron behaviors and bonding configurations. While its disadvantage includes challenges in interpreting complex spectra, the advantages of EELS in capturing fine electron details within materials are paramount for advancing our understanding of electron location.
Computational Models
Density Functional Theory
Density Functional Theory (DFT) is a cornerstone in computational chemistry, offering a powerful framework for predicting electron behaviors and properties within atomic systems. By approximating the electronic density of a system, DFT delivers insights into molecular energetics and electronic structures with remarkable accuracy. The key characteristic of DFT lies in its efficiency in handling large-scale systems and its ability to account for electron correlation effects accurately. It is a preferred choice for this article due to its applicability in studying electron interactions in diverse chemical environments. The unique feature of DFT includes its scalability and versatility in predicting electronic properties with relative ease, making it an invaluable tool for exploring electron location within complex systems.
Hartree-Fock Method
The Hartree-Fock method serves as a classical approach to understanding electron behaviors and interactions within atomic structures. By iteratively solving the SchrΓΆdinger equation for multi-electron systems, the Hartree-Fock method determines the optimal wave function for electrons, shedding light on their distribution and energies. The key characteristic of the Hartree-Fock method lies in its ability to provide accurate descriptions of electron correlations and exchange interactions, essential for studying molecular structures in detail. It is a valuable choice for this article due to its historical significance and foundational role in quantum chemistry. The unique feature of the Hartree-Fock method is its simplicity in modeling electronic configurations, although its limitations in addressing dynamic electron correlations are notable. Despite its drawbacks, the Hartree-Fock method remains a fundamental tool for researchers exploring electron location in atomic systems.
Practical Applications of Understanding Electron Location
Understanding the practical applications of electron location is pivotal in unraveling the mysteries of atomic and molecular behavior within various scientific disciplines. By delving into the precise locations of electrons, researchers and scientists can gain valuable insights into the intricacies of chemical reactions and materials science. This exploration fosters advancements in fields such as materials engineering, quantum physics, and nanotechnology while enhancing our understanding of fundamental processes governing matter.
Materials Science
Semiconductor Design
Semiconductor design plays a crucial role in modern electronics, offering a versatile platform for creating electronic devices like transistors and integrated circuits. The key characteristic of semiconductor design lies in its controllable conductive properties, making it an ideal choice for harnessing electron location in this article. The unique feature of semiconductors is their ability to switch between conducting and non-conducting states, allowing for precise control in electronic components. While semiconductor design enables the miniaturization of technology, it also poses challenges in heat dissipation and manufacturing complexity, factors that are carefully considered in this article.### Nanotechnology Innovations Nanotechnology innovations revolutionize the way we manipulate matter at the atomic and molecular levels, opening up unprecedented possibilities in various scientific fields. The key characteristic of nanotechnology lies in its ability to engineer materials with unique properties at the nanoscale, offering immense potential for applications in medicine, electronics, and energy. Within this article, nanotechnology's unique feature is its capacity to enhance electron location precision, leading to innovations such as quantum dots and nanoscale sensors. While nanotechnology brings unparalleled advancements, it also raises concerns regarding environmental impacts and ethical considerations, aspects that are highlighted and discussed in this article.
Chemical Reactions
Catalyst Development
Catalysts play a crucial role in speeding up chemical reactions without being consumed in the process, significantly impacting the efficiency and selectivity of reactions. The key characteristic of catalyst development is its ability to lower activation energies, making chemical transformations more accessible and sustainable. In this article, catalyst development is highlighted for its role in fine-tuning electron location within reaction environments, enhancing reaction rates and product yields. The unique feature of catalyst development lies in its specificity towards certain reactions, offering tailored solutions for industrial processes. Despite the advantages catalysts bring, their limitations in selectivity and deactivation mechanisms are carefully evaluated in this article.### Bond Formation Bond formation elucidates the interactions between atoms and molecules, essential for understanding the structure and properties of matter in chemical reactions. The key characteristic of bond formation is its role in creating stable chemical compounds through the sharing or transfer of electrons, influencing structural stability and reactivity. In this article, bond formation is emphasized for its impact on electron localization within molecules, dictating properties like polarity and reactivity. The unique feature of bond formation lies in its versatility to form a wide range of chemical bonds, driving the diversity of molecular structures in nature. While bond formation is fundamental in chemical processes, considerations regarding bond strength, length, and angle are pivotal factors explored in this comprehensive analysis.