Exploring the Future of Nuclear Fusion Reactors
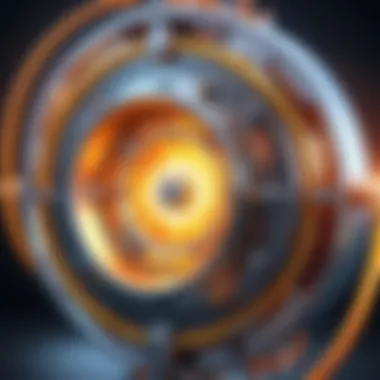
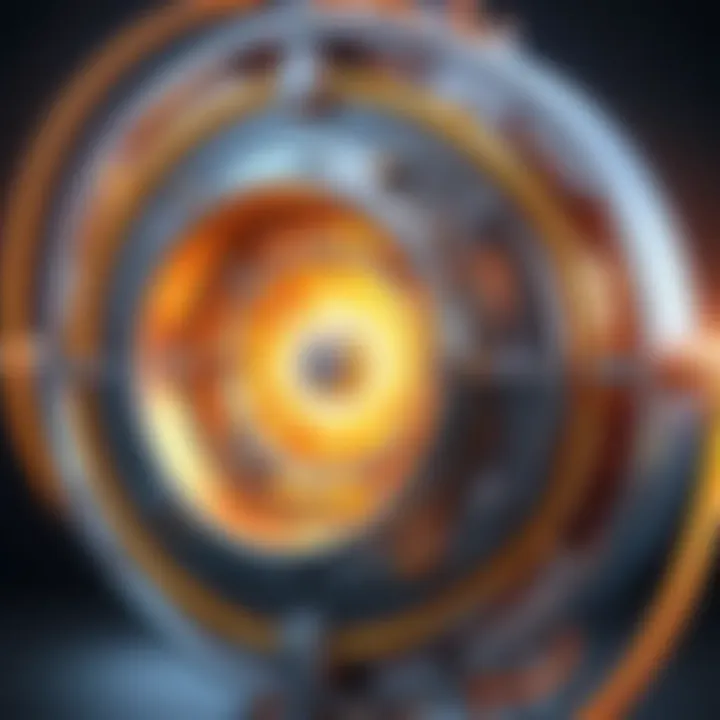
Intro
The world is at a crucial juncture when it comes to energy production. As the signs of climate change become more apparent, and traditional energy sources wane, the search for sustainable solutions is kicking into high gear. Nuclear fusion is frequently mentioned as the next big thing, touted for its potential to deliver nearly limitless energy without the harmful emissions that characterize many fossil fuels. But what exactly does this involve? What are the implications for our future? This article seeks to peel back the layers of nuclear fusion technology, providing a comprehensive guide to its principles, the innovations that drive it, and the very real impacts it could have on our society and environment.
These themes set the stage for an exploration of the current state of nuclear fusion, spotlighting the advancements and challenges that scientists and engineers are grappling with today.
Technology Insights
Latest Tech Trends
The landscape of nuclear fusion technology is changing rapidly. From the days of speculative theories to real-world applications, modern advancements have taken ideas from out of science fiction and into practical research environments.
Current initiatives include:
- ITER (International Thermonuclear Experimental Reactor) in France, where an international team is working to create a working fusion reactor model.
- SPARC, being developed by MIT and several partners aimed at realizing practical fusion energy sooner.
- Helion Energy, focusing on smaller, modular fusion systems that could potentially adjust their energy output based on demand.
These projects have ignited interest and investment, indicating a shift towards a more promising and sustainable energy future.
Innovation in Tech
Among several promising technologies, the use of plasma confinement methods stands out. Magnetic confinement and inertial confinement remain at the forefront of fusion research, with each demonstrating unique benefits and challenges. New materials capable of withstanding extreme temperatures, and advancements in superconducting magnets are also pivotal innovations propelling this sector forward.
To look at a specific curiosity, research is shifting toward advanced computing algorithms to simulate fusion reactions, potentially speeding up the trial-and-error cycle significantly.
Potential Environmental Impacts
Many regard nuclear fusion as an ultimate solution to energy needs because:
- It produces minimal waste compared to fission reactors, which utilize radioactive materials.
- The primary fuel, hydrogen isotopes, are abundant in nature and can be extracted from seawater.
- The emissions from fusion reactions do not pose a significant climate risk, as the process holds almost no carbon footprint.
"The true allure of fusion is not just in its power, but in its potential to operate with a powerful respect for the environment."
Nonetheless, potential challenges remain, such as risks involved in developing technology that operates at such extreme conditions, requiring groundbreaking engineering solutions.
At this point, it’s invaluable to widen our view that the implications of nuclear fusion go beyond just energy production; they intertwine with economic, political, and environmental dimensions that could reshape the global energy landscape significantly within the coming decades.
Understanding Nuclear Fusion
Understanding nuclear fusion is paramount in addressing the global energy crisis and the hunger for sustainable energy solutions. As our reliance on fossil fuels continues to wreak havoc on the environment, diving into the intricate workings of nuclear fusion presents a viable path forward. The essence of nuclear fusion is clear: it has the potential to provide an immense amount of clean energy that could power the world for generations.
Basic Principles of Fusion
At its core, nuclear fusion is the process by which two light atomic nuclei combine to form a heavier nucleus, releasing energy in the process. This phenomenon occurs naturally in stars, including our sun. Here, hydrogen nuclei fuse under extreme temperatures and pressures to produce helium and energy—a method that harnesses the very forces of nature.
A fusion reaction typically requires conditions that are hot enough to overcome the electrostatic repulsion between positively charged protons. Temperatures exceeding millions of degrees Celsius create a state of matter called plasma, a hot, ionized gas. To achieve this on Earth, scientists utilize advanced technology to replicate the conditions found in stellar environments.
Key Elements of Fusion:
- Fuel: The most common fuels for fusion reactions include isotopes of hydrogen, like deuterium and tritium.
- Confinement: Adequate confinement is crucial to maintain the high pressure and temperature required for fusion. This is often achieved through magnetic confinement systems or inertial confinement techniques.
- Energy Output: The energy generated through these fusion reactions is several times greater than that of fission, making it a cleaner and more efficient option for energy production.
Comparison with Nuclear Fission
While both nuclear fusion and fission release nuclear energy, they operate on fundamentally different principles. Nuclear fission involves the splitting of heavy atomic nuclei, such as uranium or plutonium, into lighter nuclei, along with a significant release of energy as well. This process is what powers most of the nuclear reactors in existence today.
Here are some notable contrasts between fusion and fission:
- Reaction Type: Fusion combines light particles, while fission breaks apart heavy particles.
- Waste Production: Fission typically produces radioactive waste that requires extensive management; fusion, on the other hand, generates minimal and shorter-lived waste.
- Safety Risks: Fission reactors have a risk of meltdown and catastrophic failure. Fusion systems, by design, are inherently safer, as any disruption or malfunction causes the reaction to stop on its own.
"Nuclear fusion may not only revolutionize our energy landscape but also pave the way for much-needed scientific advancements."
Understanding these fundamental principles help decipher the broader implications of nuclear fusion in renewable energy discourse. As we embark on refining fusion technologies, we'll explore the milestones achieved thus far and what challenges lie ahead.
Historical Context of Fusion Research
Understanding the historical context of fusion research is pivotal for grasping its significance and eventual trajectory in the energy sector. The journey of nuclear fusion isn't simply a tale of technological advancement; it's interwoven with the larger narrative of scientific exploration and inquiry. This backdrop informs not just present-day initiatives but also sets the stage for what is to come in terms of energy sustainability.
The quest for harnessing nuclear fusion has its roots embedded deep in the exploration of atomic science. In an era dominated by the search for new energy sources, researchers have historically looked toward the powerful processes that govern stars, including our own sun. Understanding fusion and its applications was no smooth sail; it required relentless efforts, discoveries, and innumerable setbacks.
“History doesn’t repeat itself, but often it rhymes.” - Mark Twain
Leaving no stone unturned, scientists ventured into the unknown to unravel the complexity of atomic nuclei. This has been crucial in framing today’s exploration into practical fusion energy. Recognizing the milestones and trials faced by pioneers in fusion research not only provides context for current technological frameworks but also illuminates the path forward, highlighting the resilience and ingenuity of the scientific community.
Early Experiments and Discoveries
The early narratives of nuclear fusion began at a tantalizing point in the late 1930s, when physicists made groundbreaking advancements in understanding the fundamental interactions of atomic nuclei. Notably, in 1938, Otto Hahn and Fritz Strassmann discovered nuclear fission, opening doors to a new way of thinking about atomic power. These discoveries set the tone, influencing subsequent research into fusion.
Synchronized with these nuclear breakthroughs were experiments focused on generating plasma and achieving controlled fusion reactions. In the 1950s, the first successful fusion reactions were achieved in the laboratory, using devices called tokamaks, which remain central to fusion research today. During this period, significant figures like Lev Artsimovich and others paved the way for future advancements in plasma confinement techniques.
Key milestones from those early years include:
- 1940s-1950s: Initial experiments revealed the conditions needed for achieving effective fusion - extreme temperature and pressure.
- 1951: The first controlled fusion reaction occurred in the United States, marking a crucial turning point for fusion research.
- 1955: The announcement of the first tokamak model in the Soviet Union highlighted the path forward.
Major Milestones in Fusion Technology
As we marched through the decades, several major milestones vividly stand out in the history of fusion technology. These milestones not only reflect the evolution of our understanding but also the various technological attepts that didn’t shy away from experimentation and learning from failure.
- ITER Initiative (1985) - This intergovernmental project aimed to promote nuclear fusion as a viable energy source. It’s one of the most ambitious scientific collaborations to date, bringing together various countries in pursuit of clean energy.
- National Ignition Facility (NIF, 2009) - Situated in the U.S., NIF has invested heavily in achieving ‘ignition’ — where nuclear fusion produces more energy than it consumes, striving towards a practical energy source.
- SPARC Project (2020) - Recent developments like the SPARC project from MIT aim to demonstrate net-positive energy from a fusion reaction by 2025, pushing the boundaries of what was thought possible.
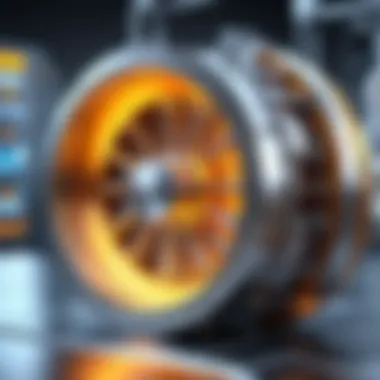
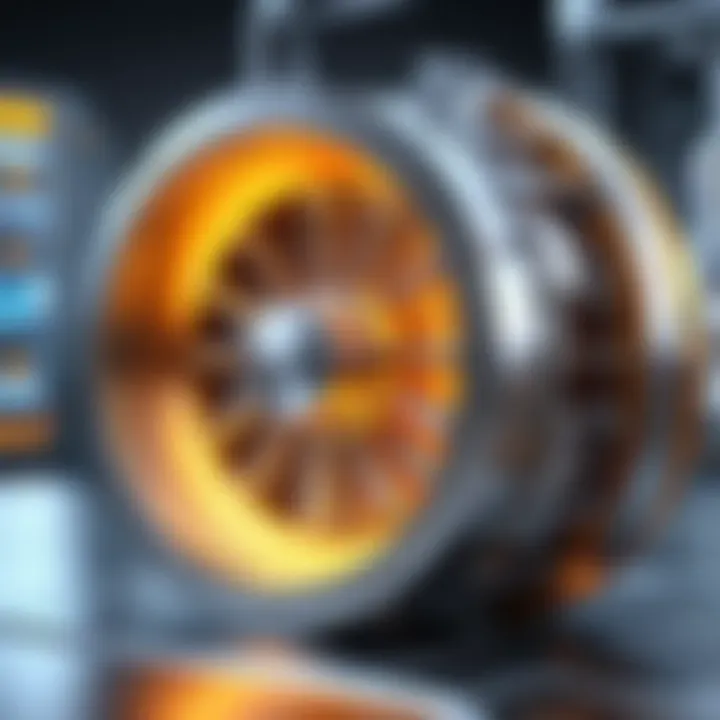
Each of these milestones encapsulates vast investment in time, resources, and intellect, underlining the complexity of fusion research. Through studying these successes and setbacks, we glean insights into the methodology of scientific advancement—a combination of collaboration, patience, and persistence.
By evaluating the historical journey of fusion research, we can appreciate its importance today. The experiences of the past not only arm present scientists with lessons learned but also instill a sense of realism about the time frame and effort required to make the leap from experimental technology to viable energy production.
Key Components of a Fusion Reactor
Understanding the key components of a fusion reactor is critical in grasping how these devices operate and the potential they have to reshape our energy landscape. The main factors influencing the functioning of a fusion reactor include plasma generation, confinement techniques, and the types of fuels utilized. Each of these elements plays a significant role in creating an environment conducive to nuclear fusion, which seeks to replicate the processes powering our sun.
Plasma Generation and Confinement
Plasma, often described as the fourth state of matter, consists of ionized gas — a mix of free electrons and ions. Generating a stable plasma is a fundamental step in the fusion process. In most reactors, this is achieved through heating techniques. Magnetic fields come into play here as well, helping to maintain the plasma's integrity. The ideal temperatures for these reactions can soar to millions of degrees Celsius, far exceeding what traditional materials can withstand. Therefore, achieving and maintaining these high temperatures without the plasma coming into contact with reactor walls presents a significant challenge.
Here are some common methods for plasma generation:
- Induction Heating: This uses magnetic fields to induce currents in the plasma, raising its temperature to fusion-viable levels.
- Microwave Heating: High-frequency microwaves are used to agitate the particles within the plasma, leading to increased kinetic energy and temperature.
Plasma stability is paramount. If the plasma cools or loses confinement, the fusion reaction can fizzle out, akin to a campfire losing its heat and becoming mere embers.
Magnetic Confinement vs. Inertial Confinement
Fusion reactors mainly utilize two methods to confine plasma: magnetic confinement and inertial confinement. Each method has its own merits and practical challenges.
- Magnetic Confinement: This technique uses strong magnetic fields to keep the high-temperature plasma stable. The tokamak reactor design, for example, employs a donut-shaped magnetic field to effectively wrap around the plasma. The goal is to prevent any contact with the reactor walls, which would rapidly cool the plasma and extinguish the fusion process.
- Inertial Confinement: In contrast, inertial confinement works through compressing a small pellet of fusion fuel (typically deuterium and tritium) using powerful lasers or other energy sources. The rapid compression heats the pellet, leading to the conditions necessary for fusion. This is a mainstay technique seen in facilities like the National Ignition Facility.
Every confinement method has its pros and cons. While magnetic confinement offers continuous operation, inertial confinement thrives on brief but concentrated bursts of energy. The choice between these two hinges on balancing efficiency, cost, and technological readiness.
Fuel Types and Their Implications
The choices made regarding fuel types impact the entire cycle of fusion energy production. The two main fuels used in nuclear fusion are deuterium and tritium. Deuterium is abundant in seawater, while tritium is radioactive and must be bred from lithium. This adds another layer of complexity to fuel management, as the reactor must ensure a continuous supply of tritium while managing the longevity and disposal concerns of the material.
Other fuel types, like helium-3 and advanced fuels based on lithium or boron, also show promise but come with their own sets of challenges, largely in terms of current technology feasibility and availability.
Implications of these fuel choices include:
- Sustainability: Fuel availability and recycling processes can influence how sustainable fusion power may become.
- Safety: Different fuels pose distinct radiation and safety concerns, needing thorough exploration during reactor design.
- Economic Factors: The cost of acquiring and processing fuel directly impacts the economics of fusion energy.
In sum, the key components of a fusion reactor intertwine in a complex dance of technology and science. Plasma generation and confinement, alongside the optimal selection of fuel types, will shape the success of nuclear fusion as a viable and sustainable energy solution.
Current Technologies in Nuclear Fusion
Nuclear fusion continues to be a beacon of hope for those yearning for a sustainable energy solution, and understanding contemporary technologies is crucial. These innovations are pivotal for moving from theory to practical applications. Each technological approach presented is a step towards harnessing the immense power of the stars, making them essential for both current research and future energy production.
Tokamak Reactors
The Tokamak reactor is perhaps the most widely known type among fusion technologies. Originating from Soviet research in the 1950s, Tokamaks utilize magnetic fields to confine plasma in a toroidal shape. This configuration is essential for maintaining the high temperatures necessary for fusion to occur. Think of it like keeping a swirling storm contained; if the storm escapes, the energy dissipates.
The recent advancements in Tokamak design, particularly seen with projects like ITER in France, have improved stability and efficiency. The approach has a promising track record; however, it is not without its quirks. The need for precision control in the magnetic confinement processes can lead to unexpected challenges, often requiring researchers to play a careful game of cat and mouse with instability issues.
Potential benefits of Tokamak reactors include:
- High energy yield from small fuel masses
- Relatively long operational lifespan compared to other methods
- Established technology with ongoing research support
However, drawbacks like complex engineering and substantial initial costs can be a hurdle for scaling up Tokamaks in the near future.
Stellarators
Stellarators, conversely, offer a distinct and innovative approach to plasma confinement without the need for a large, powerful current running through the plasma, which characterizes Tokamaks. Instead, the magnetic fields in a stellarator are created by twisted coils that allow for steady-state operation. This might sound like a simple twist in the design, but the implications are profound.
While still in the experimental phase, facilities like the Wendelstein 7-X in Germany demonstrate stellarators' potential. Their configuration allows for continuous operation, which is a significant step from the pulsed operations typical of Tokamaks. However, the intricate coil design can be hard to engineer and somewhat less energy-efficient at this stage.
Benefits of Stellarators include:
- Reduced risk of plasma instability
- Continuous operation capabilities
- Potential for lower operational costs in the long run
Despite the advantages, the complexity in construction and the current energy confinement times may slow widespread adoption until further advancements are made.
Inertial Fusion Facilities
On another frontier lies inertial fusion, where the approach hinges on compressing small fuel pellets to extremely high densities using powerful lasers or other drivers. Facilities like the National Ignition Facility (NIF) in the United States lead the charge in this realm. Inertial fusion is akin to setting off a firecracker – it requires a precise and succinct input of energy to achieve a massive output.
The goal with inertial fusion is to reach ignition, wherein the energy produced from fusion reactions exceeds the energy needed to instigate them. This has, until recently, been like chasing shadows. However, recent breakthroughs at NIF provide a glimmer of hope, as they have approached the conditions necessary to achieve this coveted result.
Key points to consider about inertial fusion include:
- Short duration of energy production events
- Potential for scalability alongside traditional fusion sources
- Significant energy releases from compact fuel sources
However, challenges such as ensuring precision in laser timing and energy delivery may limit drastic improvements in efficiency for the time being.
In sum, each fusion technology has its strengths and weaknesses, where progress in any direction contributes to the overall goal of sustainable and clean energy generation.
As we continue to explore the current technologies in nuclear fusion, it is vital to recognize their role in shaping tomorrow’s energy landscape. These innovations frame the very foundation upon which future advances can build, offering a diverse spectrum of possibilities for humankind's energetic needs.
Global Initiatives and Projects
When it comes to nuclear fusion, local efforts often pale in comparison to the global initiatives that define the landscape of fusion research. These collective projects not only serve to propel forward the scientific community’s understanding and technology but also help in pooling resources, expertise, and funding. This collaborative nature sparks innovation and fosters cooperation among nations, making it easier to tackle some of the most daunting challenges associated with developing fusion energy.
In the realm of fusion, the need for large-scale, international projects cannot be overstated. They unify scientists and engineers from diverse backgrounds and disciplines, creating a melting pot of ideas and approaches. The benefits are numerous:
- Resource Sharing: High costs of research and infrastructure are distributed among multiple stakeholders.
- Cross-Border Collaboration: Technical expertise converges from various countries, maximizing advancements in experiment designs and safety protocols.
- Consistent Funding: A broad base of funding sources can help nurtures projects in ways that would be unthinkable for isolated efforts.
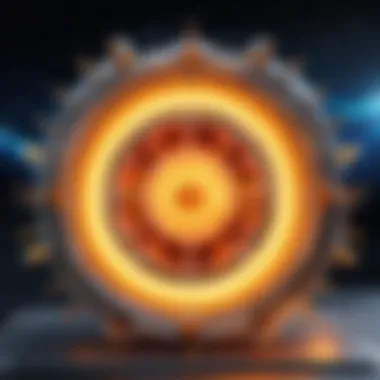
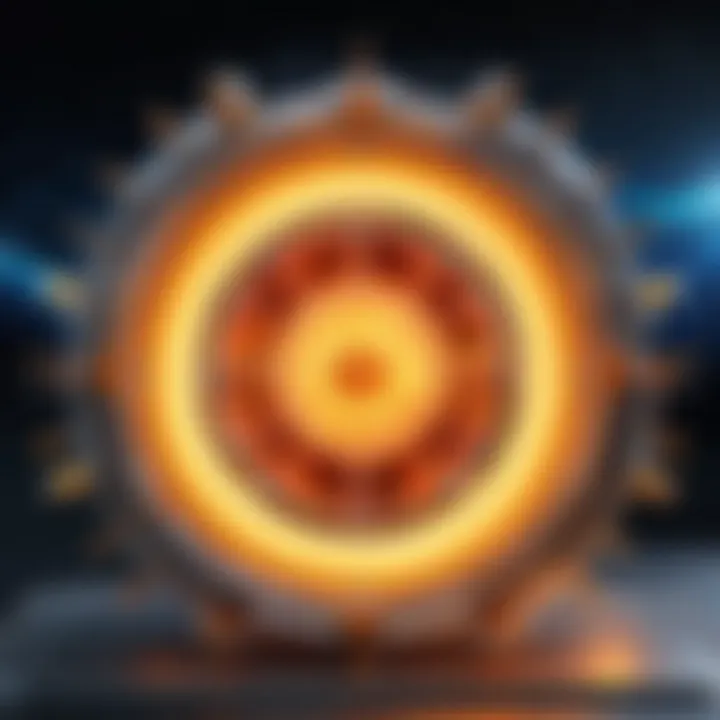
As we delve deeper, several prominent initiatives stand out, with their unique focus on expanding our knowledge of nuclear fusion.
International Thermonuclear Experimental Reactor (ITER)
ITER is perhaps the most ambitious nuclear fusion project in existence today, involving 35 nations and taking place in France. Built to demonstrate the feasibility of fusion as a large-scale and carbon-free source of energy, ITER aims not just to achieve fusion but also to lay the groundwork for future reactors. The facility is designed with cutting-edge technology and research that could redefine energy production worldwide.
One of the primary goals of ITER is to attain a plasma burn where the energy produced exceeds the energy input. This threshold is pivotal as it showcases the potential of fusion energy in a real-world scenario. The project also pushes the envelope on how devices like tokamak reactors can be operated at successively higher levels of efficiency.
“Achieving sustained plasma instabilities and efficient confinement will be the true tests for our generation of fusion researchers.”
National Ignition Facility (NIF)
The United States steps into the fusion scene with its National Ignition Facility located in California. NIF is a testament to the potential of inertial confinement fusion, aiming for breakthrough results that could pave the way for a future fusion power plant. The approach it takes is distinct—utilizing high-energy lasers to compress and heat the fusion fuel.
NIF’s mission goes beyond energy generation; it also plays a significant role in maintaining the safety and effectiveness of the nation’s nuclear arsenal. While the quest for ignition—a condition where the fusion reactions become self-sustaining—lies at the center of NIF’s operations, its technology and discoveries have vast implications for scientific research and nuclear physics as a whole.
Private Sector Ventures
In recent years, the private sector has begun to step into the arena of nuclear fusion, challenging traditional models of funding and research. Companies like Helion Energy, Tri Alpha Energy (now known as TAE Technologies), and Commonwealth Fusion Systems are making headway with innovative designs and fresh approaches. These ventures often embody a sense of agility and adaptability that is sometimes lacking in larger, government-funded projects.
The infusion of private capital and entrepreneurial spirit aims to accelerate the pace of development in fusion technologies. These companies are exploring smaller, less costly fusion projects, striving for a testable model in years rather than decades. Their endeavors show promise, suggesting that the fusion landscape is ripe for disruption and innovation.
Through these global initiatives, the dream of practical nuclear fusion is no longer some distant thought. It's evolving, driven by collective efforts that span continents and sectors, sparking hope for a cleaner and more sustainable energy future.
Challenges in Fusion Energy Development
The road to harnessing nuclear fusion energy isn't merely paved with scientific breakthroughs and technological innovations; it is also riddled with numerous challenges that could easily sideline the very vision of a sustainable energy future. Addressing these obstacles is crucial not just for researchers but for policymakers, investors, and anyone with a vested interest in the energy landscape.
Technical Constraints
When speaking of nuclear fusion, thinking about its theoretical beauty is quite straightforward. The practice, on the other hand, is fraught with complexities. Fusion requires extremely high temperatures—think millions of degrees Celsius—to initiate the process of fusing light atomic nuclei. Managing and sustaining those temperatures is where things get sticky.
One principal hurdle is plasma confinement. This involves keeping the hot plasma stable long enough to yield productive fusion reactions. Both magnetic confinement and inertial confinement are employed, yet each comes with its own set of engineering challenges. Magnetic confinement methods like those used in Tokamak reactors wrestle with the possibility of plasma instabilities, which can disrupt the process and cause energy loss.
Inertial confinement focuses on compressing fuel pellets with lasers, but precision is everything here. Any misalignment could lead to less than ideal conditions for fusion, wasting precious energy and resources.
All in all, the technical limitations are not just speed bumps; they are major obstacles that require innovative solutions to even inch closer to operational fusion reactors.
Economic Viability
The financial aspect of nuclear fusion development is another tangled web. On one hand, governments and private entities are investing millions—not to mention the promise of almost limitless clean energy. On the other hand, these projects often see cost overruns and delays that can stretch timelines into decades.
The capital expenditure required for infrastructure is staggering. Building reactors like ITER involves complex technology and collaboration among nations, but the finance needed isn’t just about construction. Operational costs, ongoing research, and maintaining public interest are just as critical.
Furthermore, the pricing of energy generated from fusion will face competition from more established renewable sources like wind and solar. Without a concrete strategy for making fusion economically attractive, it might struggle to gain foothold in a market dominated by cheaper alternatives.
Ultimately, if fusion energy is to step out of the lab and make it to the grid sustainably, it must overcome the shadow of its economic challenges.
Regulatory and Safety Concerns
Switching gears, we can't ignore the regulatory landscape surrounding nuclear energy in general. After incidents in the past involving nuclear fission, the bar is set high for safety measures, and rightly so. With fusion, the situation is often seen through an unnecessarily negative lens by the public due to misunderstandings about its safety.
For example, unlike fission, if a fusion reactor encounters problems, it doesn’t have a major meltdown risk. The fuel ()deuterium and tritium) is relatively harmless compared to uranium. However, navigating the regulatory maze to get approval for construction and operation is no small feat. Getting the go-ahead from multiple agencies, ensuring public safety, and dealing with potential environmental concerns can take years.
Moreover, as innovations emerge—such as private sector efforts in fusion—regulatory bodies are in a game of catch-up. With regulations that often haven’t matured to accommodate rapid advancements, the potential for creating a bottleneck exists.
"Handling the complexities of regulation is as much a part of fusion development as the science itself."
Environmental Impact of Fusion Reactors
The quest for sustainable energy sources has never been more pressing, and the environmental impact of fusion reactors plays a crucial role in this discussion. Unlike traditional energy sources, fusion has unique characteristics that considerably affect its ecological footprint. Understanding these implications is paramount for the broader acceptance and integration of fusion technology.
Carbon Footprint Analysis
One of the most significant advantages of nuclear fusion lies in its potential for a remarkably low carbon footprint. Fusion reactions emit minimal greenhouse gases compared to fossil fuel-based energy sources. The process primarily combines isotopes of hydrogen, like deuterium and tritium, producing helium and energy as the main byproducts—not carbon dioxide.
In practical terms, this translates to a drastic reduction in the carbon emissions associated with electricity generation. For industries and nations grappling with stringent carbon targets, this promises a viable path to achieving sustainability.
- Comparative Emissions:
When you stack the emissions from fusion against those from coal or natural gas, the numbers lean heavily in favor of fusion. In fact, the projected lifecycle emissions from fusion are nearly negligible once the reactors are in place and operational. - Construction Impacts:
It is true that the manufacturing and construction phases of fusion plants will have some emissions. Yet, these are far overshadowed by the ongoing emissions reductions once the fusion reactors are running. - Energy Efficiency:
Also, fusion reacts at incredible energies, potentially producing more energy than the minimal input needed. This efficiency aids in lowering overall emissions throughout the entire lifecycle of energy production.
In essence, investing in fusion energy could significantly shift entire economies towards carbon-neutral goals, setting a feasible target for net-zero goal deadlines on a national and international level.
Waste Management Challenges
Despite the uplifting potential of fusion energy, it's crucial to confront waste management challenges head-on. While fusion generates far less nuclear waste than fission reactors, particularly concerning long-lived isotopes, the waste generated still poses important considerations.
- Types of Waste:
The byproduct of fusion reactor operations may result in materials that become radioactive due to neutron activation. Consequently, materials employed in the reactor can become unusable and require careful storage and management. - Permanent vs. Temporary Waste:
The waste from fusion is largely short-lived compared to that produced by fission. Many isotopes decay quickly, relieving some of the long-term burdens seen in traditional nuclear waste management. Nevertheless, some materials may require handling strategies akin to those applied in fission waste. - Storage and Disposal:
Finding efficient and safe methods for disposing of this activated material is a challenge. Existing frameworks in waste management might need to adapt or evolve, bearing in mind that fusion will not eliminate waste but merely modify how it is dealt with.
Therefore, addressing the environmental impact of fusion reactors requires a balanced perspective. While fusion offers substantial promise for reducing carbon footprints, the challenges surrounding waste management and long-term strategies are hurdles that need resolution. This dialogue will play a vital role in shaping policies and developing effective strategies for a successful transition to fusion energy.
Future Prospects for Nuclear Fusion
The realm of nuclear fusion is often laced with a mixture of skepticism and hope, as the world looks towards sustainable energy solutions. The significance of understanding the future prospects of nuclear fusion cannot be understated. With the constant pressure to combat climate change and ensure energy security, the potential of fusion energy emerges not just as a scientific curiosity, but as a viable alternative that could revolutionize our energy landscape. This section aims to illuminate the key elements shaping the journey of fusion technology into the future, from optimistic predictions to the integration with other renewable sources.
Predictions for the Next Decades
As we peer into the crystal ball, predictions regarding nuclear fusion's advancement often paint an optimistic yet cautious picture. One of the most widely discussed timelines suggests that by the 2030s, we could be witnessing the first successful implementation of practical fusion reactors. This sparkling hope stems from the ongoing projects around the globe, particularly those like ITER in France. ITER aims to demonstrate the feasibility of fusion as a large-scale energy source. If successful, it could pave the way for more commercial reactors up and running in the next few decades.
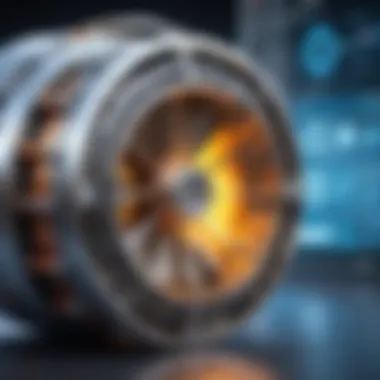
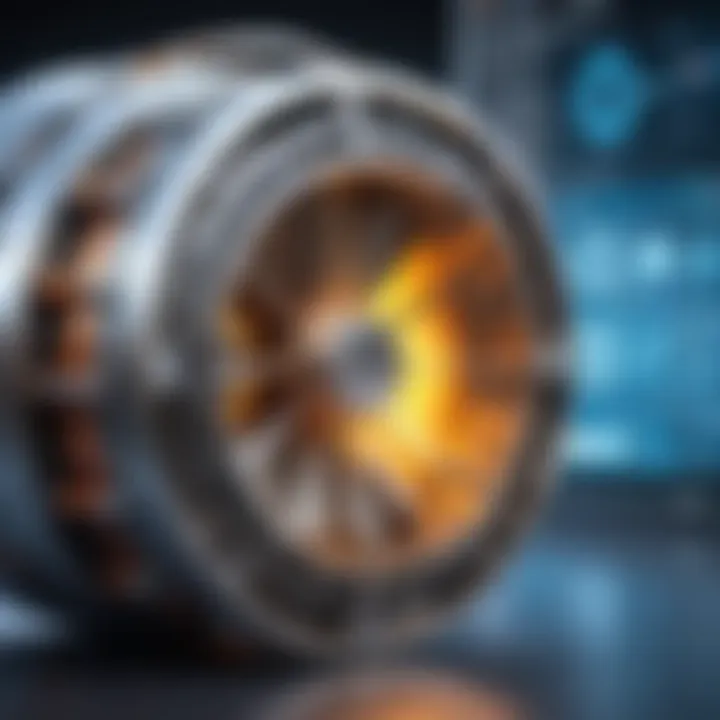
However, hitting these targets is no walk in the park. Several hurdles linger in the shadows, including maintaining plasma stability, improving energy gain, and reducing the costs associated with fusion technologies. All of these factors combine into a complex equation that needs to be solved. Here are some potential scenarios:
- Continued investment could mean breakthroughs in superconducting materials that improve magnetic confinement, enhancing overall reactor efficiency.
- Collaboration among countries may fast-track research, enabling a global fusion energy network, which could lead to shared knowledge and reduced costs.
- Alternatively, if funding and policy support falters, we may see a slower pace of development.
"To get to a point where fusion can really shine as energy source, it’s a marathon, not a sprint. We’re not looking for a quick win."
Integration with Renewable Energy Sources
The marriage of nuclear fusion with other forms of renewable energy showcases the vast potential fusion holds in the modern world. With solar, wind, and hydro already grappling with variations in supply and demand, fusion energy could provide a stable and continuous power supply. Nuclear fusion produces minimal environmental harm and generates vast amounts of energy, meaning it could work alongside renewables to create a balanced energy mix.
The synergy of fusion with renewable sources might look something like this:
- Grid Stability: Fusion could act as a stabilizing agent in energy grids, countering the unpredictability of wind and solar energy.
- Energy Storage: High-efficiency batteries could support solar and wind power, while fusion energy acts as the backbone for steady supply, especially during peak demand.
- Enhanced Energy Efficiency: Technologies associated with fusion could lead to advancements in energy technologies, improving efficiencies across the board in both old and new power plants.
Moving forward, it’s essential to foster collaborative ventures among various sectors combining fusion efforts with solar or wind initiatives. It is a gesture of optimism that could lead to a scenario where fusion energy not only complements but also enhances existing energy sources, propelling society toward a more sustainable future.
Such integration could dramatically reshape the way we view and utilize energy, shaping not just our technological future but also our ecological footprint.
Socio-economic Implications of Fusion Energy
The topic of socio-economic implications regarding fusion energy is not just a footnote in the larger narrative of energy production. It occupies a pivotal role in shaping how societies function within the global framework. Understanding how nuclear fusion can impact various socio-economic layers brings to light benefits that extend beyond just energy generation. In exploring this dimension, we can appreciate the broader implications of embracing fusion as a reliable power source.
Impact on Energy Market Dynamics
As fusion energy gears up for potential commercial use, the energy market is poised for some significant shifts. First off, as capacity builds, we could see a drop in reliance on fossil fuels which currently dominate energy sectors worldwide. This transition might not just be a matter of green enthusiasm but one involving sound economic sense.
Consider a scenario where fusion reactors become operational and start contributing to the grid. The availability of a near-limitless energy source directly affects supply and, in the long run, can drive down prices. Competition among energy providers will likely intensify, fostering innovation and efficiency. Here’s how the energy market could reshape:
- Shift in Investment Trends: With a solid future in sight, there will be an influx of capital directed towards research and development of fusion technologies.
- New Market Players: Traditional players may find themselves needing to adapt or risk obsolescence. Startups focusing solely on fusion innovation could disrupt this established landscape.
- Global Energy Equity: Countries with fewer resources might gain access to clean, affordable energy, evening the playing field on a global scale.
Fusion energy could very well rewrite the rules of engagement in the energy market, transforming dynamics that have persisted for decades.
Potential for Job Creation and Labor Market Shifts
When it comes to job creation, fusion energy presents an exciting picture, painting the landscape in vibrant strokes. The development of nuclear fusion technology involves not only scientists and engineers but also a myriad of support roles that span various domains.
A few potential avenues for job creation include:
- Research and Development Roles: As fusion research intensifies, the demand for talent in physics, engineering, and even computational modeling will surge. This isn't just limited to laboratories but also requires software development and data analytics.
- Manufacturing Jobs: Building fusion reactors involves heavy industrial processes. This can revitalize manufacturing sectors in regions where jobs have been declining, bringing new life to local economies.
- Operations and Maintenance Positions: Once operational, fusion reactors will require a workforce for ongoing maintenance and oversight, creating stable jobs over the long term.
However, it’s important to recognize that shifts in labor markets could also happen. Workers in fossil fuel industries may find their skills becoming less relevant, necessitating retraining programs to help the workforce transition into new roles. Policymakers need to be mindful of these shifts, ensuring that those affected are supported during this transition.
In summary, the socio-economic implications of fusion energy spread across various sectors, promising benefits that could reshape our energy landscape and improve lives directly. As we look ahead, it's clear that fusion energy is not just an energy promise but a comprehensive framework for socio-economic advancement.
Public Perception and Policy Framework
Understanding the dynamics of public perception alongside a robust policy framework is vital for the successful advancement of nuclear fusion technology. As it stands, nuclear fusion embodies the duality of promise and apprehension. While many view it as a potential game-changer for clean energy, misunderstandings and historical context often cloud public sentiment. Hence, a balanced narrative surrounding fusion is essential to foster wider acceptance, especially when considering its integration into the existing energy landscape.
Understanding Public Sentiment Towards Nuclear Energy
Public sentiment towards nuclear energy does not exist in a vacuum; it is a tapestry woven from historical events, media narratives, and personal beliefs. For instance, the catastrophic events at Chernobyl and Fukushima play a significant role in shaping negative perceptions. Loads of folks remember these disasters vividly and feel uneasy about anything nuclear. Thus, educating the public on the safety advances in fusion technology is imperative.
Moreover, fusion proponents must connect with communities, forming dialogues that clear misconceptions and accentuate the facts. Initiatives such as town hall meetings or educational workshops about nuclear fusion can bridge these gaps. Notably, studies have shown that informed individuals are more likely to support nuclear initiatives, recognizing fusion's potential benefits, such as reducing greenhouse gas emissions and providing a reliable energy source. Social media also offers a platform to disseminate accurate information. Just a single share on Reddit can spark conversation, helping demystify fusion for the tech-savvy audience.
"Effective communication is the backbone of public acceptance. When people understand something, fear often recedes."
Policy Recommendations for Sustainable Development
Crafting a policy framework that nurtures the future of nuclear fusion is not just about regulatory measures; it’s also about laying down a path for sustainable energy development. First and foremost, governments must commit to funding fusion research and innovation. Public-private partnerships can yield fruitful returns by combining resources from both sectors.
- Incentivizing Research and Development: Grant programs and tax credits can stimulate technological advancements and attract diverse talent. These incentives should prioritize projects with clear environmentally friendly objectives.
- Safety Regulations and Standards: Establishing clear, rigorous safety guidelines is critical. Unlike fission, nuclear fusion has a lower risk profile, but transparent standards are essential for building public trust.
- Community Engagement: Meaningful conversations at the local level should guide policies related to fusion reactors. Engaging the community early can lead to smoother integration of fusion technology into local energy grids.
- International Collaboration: Nuclear fusion transcends borders, thus fostering international partnerships can amplify research capabilities and lead to breakthroughs.
In essence, the interplay between public perception and policy must be symbiotic. A proactive approach can lay a solid groundwork for a fusion future, ensuring that the entire spectrum of stakeholders— from government entities to everyday citizens—feel a sense of investment and ownership in the remarkable potential of this technology.
The End and Final Thoughts
The exploration of nuclear fusion reactors is not merely an academic exercise, but a necessary stride towards a sustainable energy future. The issues we face today — climate change, energy scarcity, and environmental degradation — compel us to seek out innovative solutions that can deliver clean, abundant, and reliable energy. In this context, understanding the full scope of nuclear fusion's potential has significant implications.
Nuclear fusion presents a beacon of hope. As highlighted throughout the article, its foundations lie in the basic principles of merging atomic nuclei, potentially resulting in a power source that is far more efficient than its nuclear fission counterpart. There’s no denying the monumental benefits it could offer, such as reduced waste production and minimal carbon emissions. However, a balanced view must also consider the challenges we face, from technical hurdles to economic feasibility.
"The future of energy lies in our ability to harness the stars, but it requires both relentless research and public support."
In synthesizing this journey through fusion technology, several elements emerge as essential for consideration:
- Technological Viability: Continuous advancements in reactor designs, like tokamaks and stellarators, are foundation stones for future developments.
- Global Cooperation: Projects like ITER stand testament to what can be achieved when countries pool resources and share knowledge in pursuit of a common goal.
- Public Awareness and Acceptance: Education and transparency around the safety and efficacy of fusion technology are crucial for fostering an environment where innovation can thrive.
Ultimately, as we stand on the brink of potentially transformative energy solutions, it is vital that both stakeholders and the public appreciate the pivotal role that nuclear fusion may play in ensuring energy security for future generations. Each insight discussed serves as both a warning and a call to action.
Recapitulation of Key Insights
Throughout this discussion, several key insights have emerged. First and foremost, nuclear fusion is vastly different from nuclear fission. It promises a cleaner, safer means of energy production without the prolonged half-lives of radioactive waste. The technology is complex and has seen varied approaches, from traditional tokamak reactors to the more innovative stellarator designs.
Here are some essential points to remember:
- Nuclear fusion, if realized commercially, could provide nearly limitless energy from readily available fuels like deuterium and tritium.
- Current projects, such as ITER and NIF, are critical stepping stones toward the realization of functional fusion power plants.
- Fusion technology faces significant economic and technical challenges, from material resilience to the cost of construction and maintenance of reactors.
Drawing on these insights, the future landscape of energy could be shaped dramatically by how effective we can be in overcoming the obstacles presented.
Call for Continued Research and Innovation
As we ponder the myriad possibilities presented by nuclear fusion, it becomes transparent that continual research and innovation are paramount. The road forward is strewn with risks and uncertainties, yet that often is the precursor to groundbreaking discoveries. Governments, universities, and private entities must dedicate resources to advance our understanding and capabilities in this area.
A strategic focus should include:
- Investment in Research: Allocating funds to explore new materials that can withstand extreme conditions encountered in fusion reactions.
- Interdisciplinary Collaboration: Fostering partnerships among physicists, engineers, and environmental scientists to enhance the development of fusion reactors.
- Public Policies Supporting Innovation: Crafting frameworks that provide incentives for private investment into fusion technologies can accelerate progress.