Exploring the Smallest Supercomputer: Design and Impact
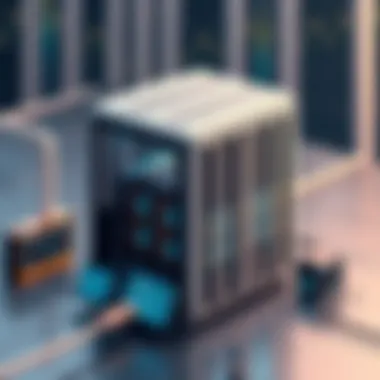
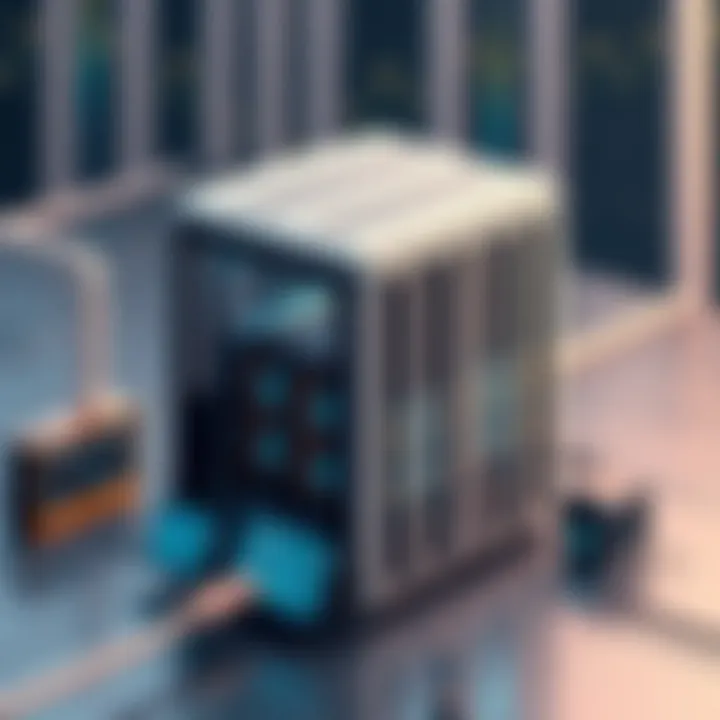
Intro
The rise of supercomputers has undeniably changed the landscape of computational capabilities, pushing the envelope on what technology can achieve. However, as these towering beacons of processing power continue to dominate headlines, an intriguing trend emerges in the form of the smallest supercomputers. These compact systems, often overshadowed by their larger counterparts, provide unique specifications and a myriad of applications that deserve attention. This exploration aims to dissect the concept of these diminutive giants, shedding light on their functionality, design, and how they are making waves across various industries.
In this journey, we will dive into Technology Insights, focusing on cutting-edge reasons behind the surge of interest in these small supercomputers. Expect to uncover groundbreaking innovations that challenge traditional notions of computing power.
Technology Insights
Latest Tech Trends
As the landscape of technology continuously evolves, the spotlight has shifted towards the miniaturization of computing systems. Smaller, more powerful machines have seen an upsurge due to increased demand for processing power in compact formats. Notable trends include the integration of ARM architecture in supercomputing, which allows for lower energy consumption while maintaining high performance. This has paved the way for devices that not only take up less space but also significantly reduce operational costs.
Innovation in Tech
Innovation doesn’t only refer to larger capacities or faster speeds; it also incorporates energy efficiency and accessibility. The smallest supercomputers exemplify this shift by making complex computations manageable for a wider variety of users, including small businesses and educational institutions. Utilizing systems such as the Raspberry Pi, enthusiasts and professionals alike are building low-cost supercomputing solutions that previously seemed like distant fantasies. This democratization of tech means a broader pool of talents can leverage advanced computing without breaking the bank.
Comparing Performance
While a traditional supercomputer may boast thousands of cores and pets of memory, the smallest supercomputers show that sometimes less is more. Consider:
- NVIDIA Jetson Nano: Compact yet capable, it provides GPU-integrated computing power perfect for AI applications.
- Beowulf Clusters: These distributed computing solutions leverage multiple smaller machines to achieve supercomputing capabilities.
In competitive scenarios, it's not just about raw power; it's also about the ability to iterate faster in a flexible environment, which smaller supercomputers typically facilitate.
"The future of supercomputing lies in its accessibility and efficiency, rather than sheer size."
- Anonymous Tech Expert
Through dissecting these trends, we can see a clear narrative: smaller doesn't mean lesser.
Implications Across Industries
The applications of these compact systems span across multiple domains:
- Healthcare: Small supercomputers are used for analysis of complex medical imaging and data.
- Research: Universities are pushing boundaries with projects that require intense computational power, usually within limited budgets.
- AI Development: Startups can now experiment with machine learning models without needing vast infrastructure.
As we examine the smallest supercomputers, the impact made on these industries and others illustrates the broader revolution that is taking place. They're not just fascinating gadgets; they represent a fundamental shift in our approach to computing.
In the next sections, we will delve deeper into the design features and technical specifications of these devices, ensuring a comprehensive understanding of their roles in today’s tech landscape.
Understanding Supercomputers
The importance of understanding supercomputers lies in their pivotal role in advancing technology. These machines are the backbone of high-performance computing, capable of processing immense data sets at lightning speeds. Their capabilities extend beyond traditional computing limits, creatively solving complex problems in fields like climate modeling, astrophysics, and intricate simulations in engineering. As we wade deeper into the era of AI, the relevance of supercomputers becomes ever clearer—they enable innovations that shape the very framework of our digital existence.
However, as we look at the smallest supercomputers, a new chapter in this field requires equal attention. It's not just about power anymore; it’s the combination of performance and compact design that sparks interest. Compact supercomputers present unique challenges and opportunities. This emerging niche offers benefits, particularly concerning energy efficiency, cost-effectiveness, and increased accessibility to smaller institutions.
In short, comprehending supercomputers lays a foundation for exploring their smaller counterparts, providing essential insights into how miniaturization can bring sophisticated computing capabilities within reach of diverse sectors—from academic institutions to industrial applications.
Definition of a Supercomputer
A supercomputer is typically understood as a computing machine that excels in processing capability. It operates at speeds measured in trillions of calculations per second, known as TeraFLOPS (Floating Point Operations Per Second) or even PetaFLOPS for more advanced systems. This staggering level of power enables the handling of immensely complex tasks, such as real-time data analytics, weather forecasting, and drug discovery. These machines harness thousands of processors working simultaneously, displaying performance that dwarfs conventional computers.
The Evolution of Supercomputers
The journey of supercomputers has been anything but linear. They began as colossal machines filling entire rooms, relying on vacuum tubes, and later transistors, before transitioning to high-density integrated circuits. Each step in this evolution has not only increased computational capacity but also shifted their usability.
In the early days, supercomputers were tools of governments and universities, primarily used for groundbreaking research. Over time, the corporate world recognized their potential; industries like automotive and aerospace adopted them for simulations and design tasks. Currently, we are witnessing a shift towards smaller, more efficient supercomputers, which can be found in various settings including small research labs and corporate offices.
The Criteria for Measuring Supercomputer Performance
Performance is king in the realm of supercomputers, and specific criteria help differentiate the leading models. Some key aspects include overall speed, efficiency, and capacity.
Top List
The Top 500 List stands as a testament to the rapid advancements in computational prowess. Released biannually, this ranking catalogues the fastest supercomputers globally, allowing for a clear comparison of technological achievements. The main characteristic that sets the Top 500 apart is its focus on performance through FLOPS, making it a reliable indicator of a system's capability. Its significance for our article lies in showcasing how performance benchmarks have evolved over time and how smaller supercomputers can not only fit into this list but can also redefine the traditional standards.
The unique feature of the Top 500 list is its credibility and wide recognition among researchers and technologists. However, it does pose an inherent downside: it often highlights existing giants while new players, especially smaller systems with niche applications, may struggle to make their mark.
FLOPS Benchmarking
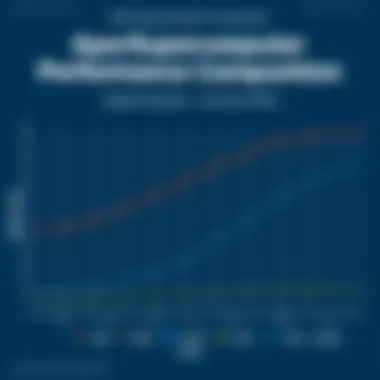
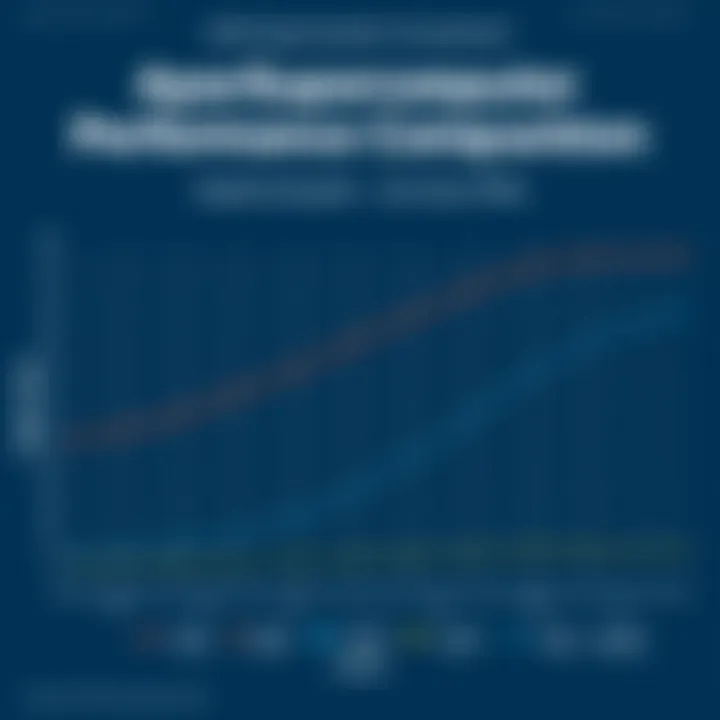
FLOPS benchmarking measures a computer's performance specifically in floating-point calculations, which are essential for tasks like scientific simulations. This characteristic makes FLOPS an influential metric, often cited in discussions about computational speed. Its weight in this article comes from the fact that as technology has progressed, smaller supercomputers are now also making strides in matched performance, challenging the very definitions used for classification.
The unique aspect of FLOPS testing is its direct relation to real-world applications, informing users on how a system will perform in data-intensive tasks. On the flip side, relying solely on FLOPS can misrepresent the overall capacity of a supercomputer, as other factors like memory bandwidth and energy efficiency are also crucial in determining usability.
Defining the Smallest Supercomputer
The small supercomputer embodies a fascinating juxtaposition of size and performance. Understanding this concept is crucial as it addresses the need for compact computing solutions without compromising on power. In a world increasingly leaning towards efficiency and portability, small supercomputers represent a vital development in technology that meets various demanding requirements. From scientific research to industrial applications, these supercomputers serve an indispensable role, allowing organizations to harness advanced processing capabilities while managing space and energy concerns.
The Concept of Size in Computing
Physical Dimensions
Physical dimensions are a critical parameter in defining what constitutes a small supercomputer. Generally, these systems occupy significantly less space compared to traditional supercomputers, making them suitable for environments with space constraints like laboratories or small offices. One of the key characteristics of the physical dimensions of small supercomputers is their ability to fit into standard server racks, which can be a game changer in settings that can’t afford to lose a lot of room. This feature not only saves valuable space but also eases transportation and installation, making them an attractive option for many enterprises.
A notable aspect of the size consideration is the design philosophy. The miniaturization of components means that the systems can integrate advanced technologies without sprawling footprints. While small size is beneficial, it also comes with limitations. For instance, densely packed components can lead to heat management issues, which must be addressed carefully to maintain system reliability.
Processing Power vs. Size
The relationship between processing power and size is complex but critical to understanding the capabilities of small supercomputers. Typically, the main objective in developing these systems is to achieve a balance where reduced size does not entail a significant reduction in computational performance. A characteristic feature of these systems is their ability to deliver high performance per watt of energy consumed. This makes them particularly advantageous in situations where power efficiency is paramount.
Interestingly, as the size shrinks, the advancements in architecture mean that we can achieve higher processing power in more compact forms. However, this also leads to unique challenges. For instance, while some small supercomputers can match the performance of larger counterparts in specific tasks, there may be scenarios where they lack the sheer power needed for heavy computations that require vast resources. Therefore, organizations must carefully weigh the pros and cons of utilizing these machines based on their specific computational needs.
Historical Milestones in Small Supercomputers
The journey of small supercomputers is one marked by significant milestones that illustrate technological advancement. Early developments in parallel processing allowed smaller organizations to leverage computational power previously reserved for larger enterprises. One of the more notable breakthroughs was the introduction of the Beowulf cluster architecture, which made it feasible to interconnect commercial off-the-shelf computers for supercomputing tasks, paving the way for the rise of compact supercomputers.
In more recent years, companies such as NVIDIA have introduced powerful GPUs that fit into smaller form factors, contributing significantly to the ongoing evolution in this space. Such progress has allowed researchers and industry professionals alike to run simulations and data analyses that were once thought impossible on a smaller scale. These milestones stand evidence of how innovations in technology have continually redefined what is achievable for small supercomputers, establishing their relevance in various fields
"The rise of small supercomputers reflects a broader trend in technology where functionality is increasingly sought in smaller packages, without sacrificing performance."
Through examining these developments, it becomes evident that small supercomputers are not merely diminished versions of their larger peers; they are distinct entities that offer unique capabilities suitable for a broad spectrum of applications.
Technical Specifications of the Smallest Supercomputers
The realm of supercomputing is often dominated by ideas of immense size and power, yet the smallest supercomputers are stepping into the limelight, challenging conventional perceptions. Examining their technical specifications sheds light on not only their capabilities but also their roles in various applications. The intricate interplay of architecture, processing power, and memory does not just define these machines; they depict a broader shift in computing practices. Their benefits, from portability to energy efficiency, revolutionize how we conceive of high-performance computing in today’s world.
Architecture and Design
Chipset Innovations
When discussing chipset innovations, one cannot overlook the pivotal role that new materials and designs play in shaping the capabilities of small supercomputers. One standout is the shift from traditional silicon to gallium nitride (GaN) chips. GaN offers immense benefits, particularly in heat dissipation and energy efficiency. This characteristic makes it a popular option, especially when space and thermal output are significant concerns, like in compact devices.
An important feature of modern chipsets is their ability to integrate numerous processing cores onto a single chip. This innovation leads to increased parallel processing, meaning these supercomputers can handle multiple tasks simultaneously. This is particularly advantageous when processing large datasets, often encountered in scientific research. However, there exists a caveat; with more cores, programming complexity increases, requiring advanced algorithms to effectively harness this power.
Modular Configurations
Modular configurations are another key aspect of small supercomputers that enhance their versatility. Unlike traditional monolithic designs, small supercomputers often employ a modular approach, allowing for incremental upgrades without replacing entire systems. This feature is not just a matter of convenience; it significantly extends the lifespan of the hardware and helps organizations manage technological obsolescence more effectively.
A defining characteristic of modular setups is their scalability. This adaptability is crucial for environments where needs can change rapidly, such as in research institutions or startups. They can start small with basic components and then scale up as necessary, making them a pragmatic choice. However, potential downsides do exist—coordinating multiple modules requires precise compatibility and can introduce challenges during integration.
Processing Power
The processing power of small supercomputers is a central element in their operational integrity. It is powered by advancements in multi-core and many-core processors. These chips, featuring map architecture, provide significant computational throughput without corresponding increases in physical size.
The rise of specialized processing units, such as Graphics Processing Units (GPUs) and Tensor Processing Units (TPUs), further drives this field forward. These units are optimized for parallel tasks, making them exceptional for tasks involving artificial intelligence and machine learning. Their integration into compact systems marks a notable milestone in the operational abilities of small supercomputers, allowing them to perform functions previously relegated to much larger counterparts.
Memory and Storage Options
Memory and storage choices for these small supercomputers are critical, impacting performance and efficacy. Solid State Drives (SSDs) have become the go-to option for many, given their superior speed compared to traditional hard disk drives. This performance is vital for tasks that require quick data retrieval and processing, particularly for large-scale simulations or real-time data analysis.
On the memory side, advancements in DDR (Double Data Rate) chips facilitate higher bandwidth while maintaining compact form factors. Furthermore, technologies such as HBM (High Bandwidth Memory) exemplify the trend toward memory solutions that marry speed with efficiency, offering attractive dividends for small supercomputers, particularly in applications requiring heavy computational lifting. However, higher-performance options can come with a price tag that not all organizations might be ready to meet.
In summary, the technical specifications governing small supercomputers reflect a landscape of innovation where size no longer dictates power. Continuous advancements in architecture, processing capabilities, and memory options pave the way for these machines to redefine what high-performance computing means. Their role in exapnding the reach—whether in industry, academia, or innovative startups—illustrate a promising horizon for compact computing solutions.
Comparing Small Supercomputers to Traditional Models
In the realm of computing, the comparison between small supercomputers and traditional models is not just a mere academic exercise; it’s a pivotal narrative that reshapes our understanding of computational power and its applications. Small supercomputers offer distinct advantages that can vastly differ from what traditional systems provide. Grabbing attention within academia and industries alike, this exploration showcases how these compact yet powerful devices are finding their way into various sectors.
Performance Metrics
Processing Speed
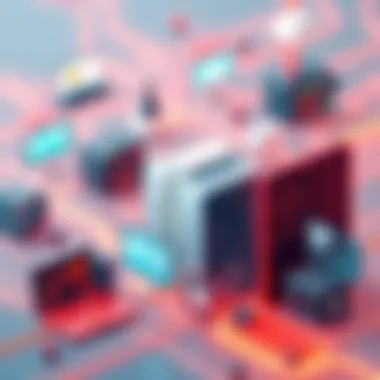
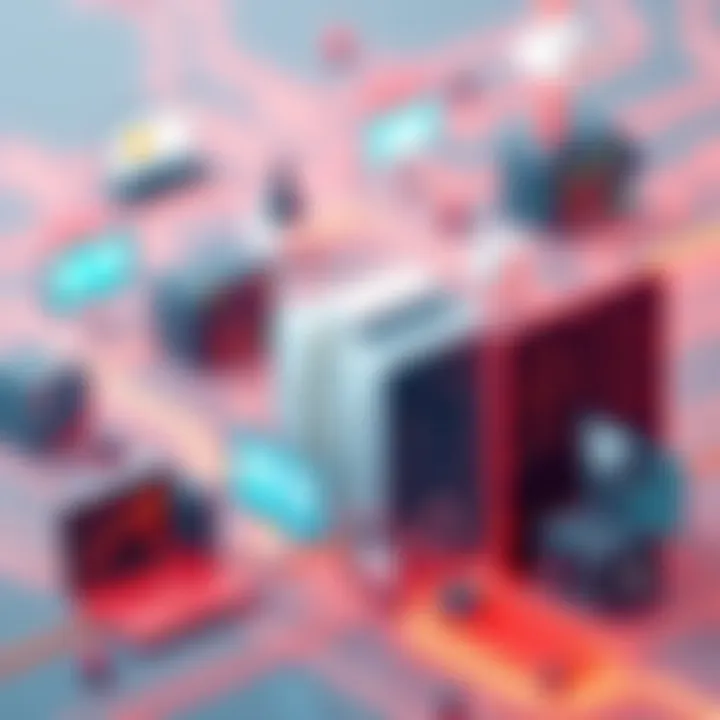
When diving into the processing speed of small supercomputers, it’s clear that this characteristic is what often drives innovation and excitement in computation. The small form factor doesn’t compromise the speed many expect from full-sized supercomputers. In many cases, they can match or even excel in certain tasks due to their architecture, which can be optimized for speed.
For example, utilizing cutting-edge chipsets like NVIDIA’s Jetson series, small supercomputers can process massive datasets rapidly. The key takeaway here is that processing speed is no longer tied to the size of the machine. This characteristic allows small supercomputers to seamlessly integrate into workflows previously only tackled by larger systems.
However, a notable downside is heat production; as the speed ramps up, so does the need for effective cooling solutions, which can be tricky in smaller packages.
Energy Consumption
Energy efficiency is another major player in the game of small versus traditional supercomputers. These devices are designed with an acute awareness of energy usage, often consuming significantly less power relative to their larger counterparts.
A prime example here is IBM’s Summit, which while powerful, requires an immense energy supply. Conversely, small supercomputers capitalize on low-energy designs that enable the execution of complex calculations without breaking the bank. This becomes crucial especially in sectors where power costs can skyrocket.
The unique feature of energy consumption is its correlation with operational costs and environmental impact. Small supercomputers not only provide high performance but do so with a lighter ecological footprint. Nevertheless, it’s crucial to balance this with the performance needs of certain applications where energy efficiency might come at a cost of processing power.
Use Case Scenarios
Academic Research
In the field of academic research, small supercomputers serve instrumental roles. They allow researchers to conduct simulations and data analysis which were previously only feasible in large-scale environments. The primary characteristic making them beneficial is their versatility: they can be used for everything from bioinformatics to serious computational physics without occupying an entire lab space.
A 200-core small supercomputer can effectively analyze genetic data sets, enabling breakthroughs that might take traditional systems days to process, minifying the timeline for research outcomes.
However, while they offer flexibility, the downside is that for particularly large datasets or highly complex computations, they may hit performance ceilings more quickly than larger systems, necessitating thoughtful project planning.
Corporate Applications
Corporate applications present another arena where small supercomputers shine. They enable fast data processing in fields such as finance, marketing analytics, and product design, making them invaluable tools for decision-making. A key characteristic of these systems in corporate landscapes is their ability to provide real-time insights, allowing companies to adapt strategies swiftly.
For instance, in retail, a small supercomputer can crunch customer data and trends, leading to more effective inventory management or personalized marketing initiatives.
One downside to consider is scalability; as a company grows, they might find themselves needing more computing power, which could lead to upgrading costs or integration hurdles with existing systems.
In summary, understanding how small supercomputers compare with traditional models highlights their unique positions and capabilities in modern computing landscapes—balancing speed, energy use, and practical applications.
Applications of Small Supercomputers
The applications of small supercomputers mark a significant shift in how computing power is perceived and utilized across various sectors. These sophisticated yet compact machines are not just smaller versions of their larger counterparts; they bring unique capabilities and efficiencies that open doors to innovative solutions in several fields, including science, industry, and healthcare. Understanding these applications provides insight into the practical implications of supercomputing in today's world.
In Scientific Research
Data Analysis
Data analysis stands as a vital aspect of scientific inquiry, and small supercomputers are revolutionizing this process. In research environments where massive datasets are common, the ability to swiftly analyze and derive meaningful insights is crucial. Small supercomputers, with their powerful processing abilities, enable researchers to handle complex datasets without the confines of traditional, bulky systems. Their compact size means they can be used in smaller labs or even brought to field locations, making them a beneficial choice for researchers aiming to conduct analyses remotely.
A key characteristic of small supercomputers in data analysis is their energy efficiency. They balance performance with lower energy consumption, making them attractive for long-term projects where cost is a concern. The unique feature of these systems is their ability to run advanced algorithms in real time, significantly speeding up the process of data interpretation.
However, adopting small supercomputers for data analysis is not without challenges. The limited processing capabilities compared to larger models could potentially hinder performance for extremely large datasets. Yet, for many applications, their advantages far outweigh these potential downsides.
Simulations
Simulations have taken on new life with the advent of small supercomputers. These systems allow researchers to model complex phenomena with unprecedented speed and efficiency. Fields like meteorology, molecular biology, and physics heavily rely on simulations to test theories or predict outcomes. Small supercomputers can run simulations that would typically require vast resources, making them a popular choice in research facilities focused on maximizing productivity within budget constraints.
A standout feature of small supercomputers in simulations is their ability to execute high-fidelity models. This means researchers can achieve more accurate results faster, leading to better informed decisions and breakthrough discoveries. Yet, one must consider the potential trade-offs; while they excel in smaller-scale simulations, there may be restrictions in tackling extremely detailed or large-scale models. Nevertheless, these machines continue to redefine the potential of computational research.
In Industry
Manufacturing Processes
In the realm of manufacturing, small supercomputers are proving to be game-changers. They facilitate the optimization of production processes by analyzing workflows in real time and adjusting variables to increase efficiency. Their strength lies in their capability to process vast amounts of data generated on the factory floor without needing an expansive physical footprint. This adaptability makes small supercomputers an excellent asset for manufacturers looking to streamline operations.
A characteristic that sets these systems apart is their modularity; they can be integrated seamlessly into existing manufacturing setups. The main advantage is that businesses can improve their processing speed and data accessibility without the need for extensive infrastructural changes. On the downside, some may find that their lower processing power limits the complexity of analysis, but many manufacturers have found that these systems meet their needs effectively.
Circuit Design
Circuit design is another area where small supercomputers shine. The design of modern electronic components demands considerable computational power, and small supercomputers fit this need perfectly. Their high-speed processing capabilities allow engineers to simulate electronic circuits efficiently, testing various designs before physical prototypes are created. This significantly reduces costs and time spent in the design phase, a key advantage in a fast-paced industry.
Moreover, the compact nature of these supercomputers makes them easy to implement in design offices or labs. A notable feature is their ability to perform parallel processing, which is crucial when working with complex circuit designs. However, the trade-off for utilizing these machines can be that they may not always match the robustness of larger systems for highly intricate designs, though many find them sufficiently equipped for typical tasks.
In Precision Medicine
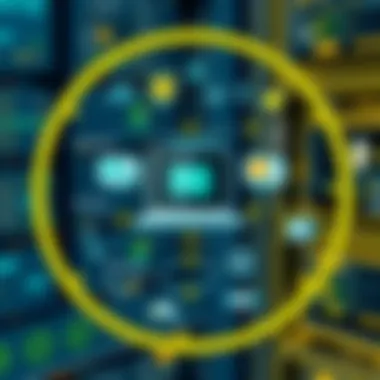
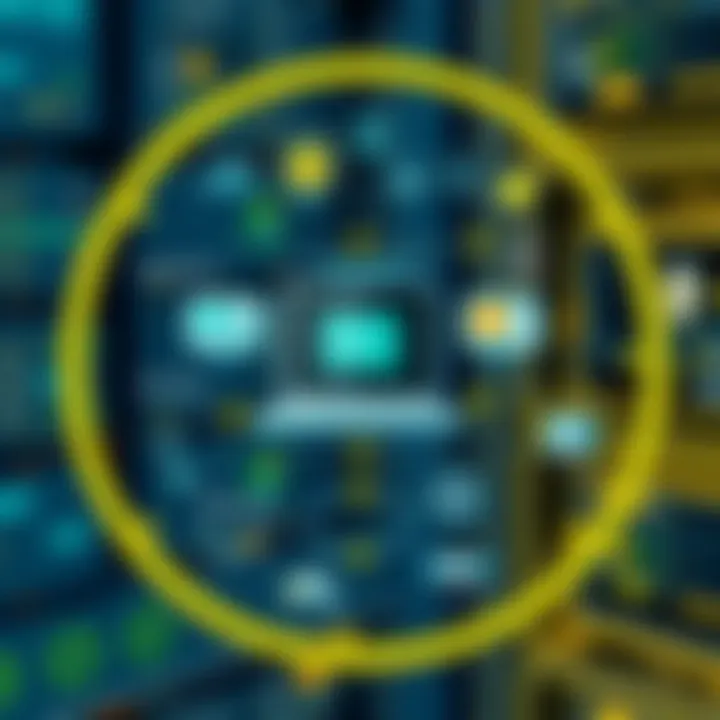
Precision medicine, which aims to tailor medical treatment to individual characteristics, is increasingly relying on small supercomputers. These systems are adept at processing massive datasets originating from genomics and patient health records which helps in formulating more personalized treatment plans. The small supercomputers can handle the intricate calculations required to analyze genetic information and predict disease risk accurately.
The key to their success in this sector lies in real-time processing capabilities, allowing healthcare professionals to make informed decisions faster. A unique aspect of using small supercomputers in precision medicine is their ability to maintain patient confidentiality while managing large volumes of sensitive data. On the flip side, there may be concerns about data security and integration with existing healthcare infrastructures. Despite these challenges, the potential benefits for personalized healthcare solutions are enormous.
Challenges in Small Supercomputer Development
In recent years, small supercomputers have emerged as powerful players in the world of computing. However, their development is not without hurdles. Understanding the challenges faced in this domain highlights both the complexity and potential of these compact systems. Addressing these challenges is critical as they can influence performance, usability, and ultimately, the adoption of small supercomputers across various sectors.
Heat Management
One of the foremost challenges in developing small supercomputers revolves around heat management. As these machines pack an astonishing amount of processing power into a diminutive size, they generate significant heat. When the electrical components work overtime, the temperature can rise quickly, potentially leading to overheating and component failures.
To mitigate this, engineers have turned to innovative cooling solutions. For example, liquid cooling systems are being integrated into smaller models. These systems circulate coolant through the hardware, absorbing heat more efficiently than traditional air cooling methods. Another approach involves the use of microfluidic cooling, where tiny channels carry coolant in close proximity to heat sources, keeping temperatures stable. This kind of design trade-off is essential, as balancing cooling efficiency with space constraints can be a tricky dance.
"Heat management doesn’t just protect hardware, it ensures sustained performance, enhancing the system's reliability and lifespan."
Scalability Issues
Scalability is precisely where small supercomputers might fall short compared to their larger counterparts. When talking about scalability, it’s not only about adding more hardware but also about whether the system can grow in a proportional manner, maintaining effective performance levels. The compact architecture of small supercomputers can limit the capabilities to expand or upgrade. Limited processing nodes may constrain simultaneous tasks, affecting their overall throughput.
Larger systems can easily reposition and adapt their hardware as demands scales up. In contrast, if a smaller supercomputer is saturated, it might necessitate almost a complete redesign to incorporate next-generation technologies. This can place a hefty price tag on what seems a relatively straightforward task.
Integration with Existing Technology
Finally, integrating small supercomputers with existing technology presents challenges that must be navigated carefully. Many industries have legacy systems in place, which may not be compatible with the latest compact supercomputing technology. Ensuring these systems communicate seamlessly becomes paramount for organizations. A significant amount of modification might be necessary for coherent integration.
It's essential that developers consider not just the performance of a small supercomputer, but also its adaptability into environments outfitted with older systems. This is a multi-faceted issue with performance, cost, and timeline implications, all of which can dramatically affect an organization’s decision on whether to invest in these systems.
In summary, while the market for small supercomputers is burgeoning, significant challenges remain in their development. Tackling heat management, addressing scalability issues, and ensuring smooth integration with existing technologies will be critical for their future uptake in various arenas.
Future of Small Supercomputers
The future of small supercomputers is not just a whimsical notion but a growing realm that is crucial in today’s technological landscape. As industries increasingly rely on high-performance computational capabilities, the importance of maintaining compact, efficient, and powerful systems cannot be overstated. These miniature marvels present unique opportunities and challenges, particularly with the advent of emerging technologies and evolving market demands.
Emerging Technologies
Quantum Computing
Quantum computing stands out as a pivotal development in the realm of computational technology. This approach leverages the principles of quantum mechanics to perform complex computations at unprecedented speeds. Unlike classical computers that process information in bits, quantum computers utilize quantum bits, or qubits, which can exist in multiple states simultaneously. This key characteristic enables quantum systems to tackle problems that are notoriously difficult for traditional computers, such as large-scale simulations and cryptographic challenges.
One significant advantage of quantum computing lies in its potential to exponentially accelerate data processing. Researchers are already exploring its applications in drug discovery, where vast quantities of molecular data can be analyzed in a fraction of the time it would take a classical supercomputer. However, it’s important to be aware of the disadvantages too, such as the current instability of qubits and the high costs associated with developing reliable quantum systems. Thus, while it's a powerful choice for tackling complex problems, the maturity of this technology still requires careful consideration.
Edge Computing
On the other hand, edge computing is gaining traction as a game-changer for small supercomputers, particularly in scenarios requiring real-time data processing. By processing data closer to where it is generated, this technology reduces latency and bandwidth usage, making it an appealing option for industries that require instantaneous feedback, such as autonomous vehicles and smart cities.
The defining characteristic of edge computing is its ability to streamline operations without necessitating constant communication with centralized data centers. This unique feature not only enhances performance but also contributes to energy efficiency, as less power is consumed in data transmission. However, integrating edge computing with existing infrastructures can pose challenges, such as security concerns and the need for robust software solutions. Nonetheless, its benefits make it an appealing focus for the future of small supercomputers.
Potential Market Growth
The market for small supercomputers is poised for remarkable growth in the coming years, driven by escalating demands in various sectors. As organizations increasingly prioritize data-driven decision-making, the scalability and capability of these compact systems are becoming more crucial. There’s a definite need for solutions that offer high processing power without the physical space requirements of larger systems.
Key Factors Influencing Growth
Some key elements influencing this market expansion include:
- Advancements in a device miniaturization: As technology progresses, miniaturizing components makes it possible to build powerful machines in smaller formats.
- Increased reliance on AI and machine learning: These fields require intensive computational resources, which small supercomputers can efficiently supply.
- Focus on energy efficiency: As businesses strive for sustainability, the energy-efficient design of small supercomputers is becoming more attractive.
Culmination
In wrapping up our exploration, it becomes clear that understanding small supercomputers isn’t just a matter of grasping their technical specifications or operational capabilities. There are critical takeaways that illuminate their burgeoning significance in the tech landscape. These mini marvels harness immense processing power packed into compact forms, setting a new stage for innovation across diverse industries.
Summarizing Key Insights
To distill the essence of what we've discussed:
- Size vs Performance: The very notion of a supercomputer is being redefined. Small supercomputers offer incredible performance, challenging the age-old assumption that bigger means better.
- Efficiency: With their innovative designs, these machines consume less energy while maintaining proficiency, making them not just an alternative but a more sustainable choice in some contexts.
- Applications: From academic research to industrial applications, the versatility of small supercomputers is a game changer. Their capacity for handling complex data analysis and simulations has broad implications for sectors like healthcare and manufacturing.
- Future Potential: The trajectory we’re on suggests a rise in dependence on smaller, modular systems as technology continues to advance at breakneck speed.
Overall, small supercomputers emerge as critical players in the evolving tech ecosystem, where adaptability and efficiency reign supreme.
The Role of Small Supercomputers in Future Computing
Looking ahead, small supercomputers are set to play a pivotal role in the next wave of computing innovation. Several factors contribute to this:
- Quantum leaps in technology: As quantum computing gains ground, small supercomputers could serve as the ideal testing grounds. They’ll offer researchers a refined platform for experimentation at reduced costs and smaller footprints.
- Edge Computing: The shift towards edge computing suggests that tasks requiring immediate data processing will benefit greatly from the nimbleness of these compact systems. Their deployment in locations closer to data sources minimizes latency, a paramount factor in real-time applications.
- Ecosystem Integration: The rise of IoT devices and AI applications call for high-performance computing that doesn't necessarily need large infrastructures. Small supercomputers can bridge that gap by working in tandem with these technologies.
"The compact design and powerful capabilities of small supercomputers symbolize a future where excellence is no longer measured by size, but by what you can accomplish within limited resources."