Exploring the Future of Nuclear Fusion Reactors
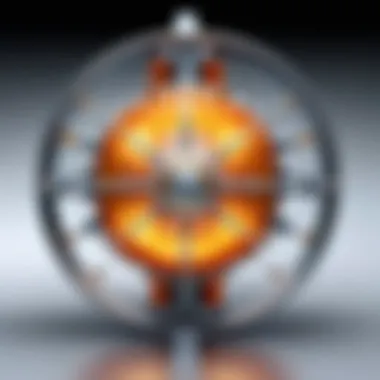
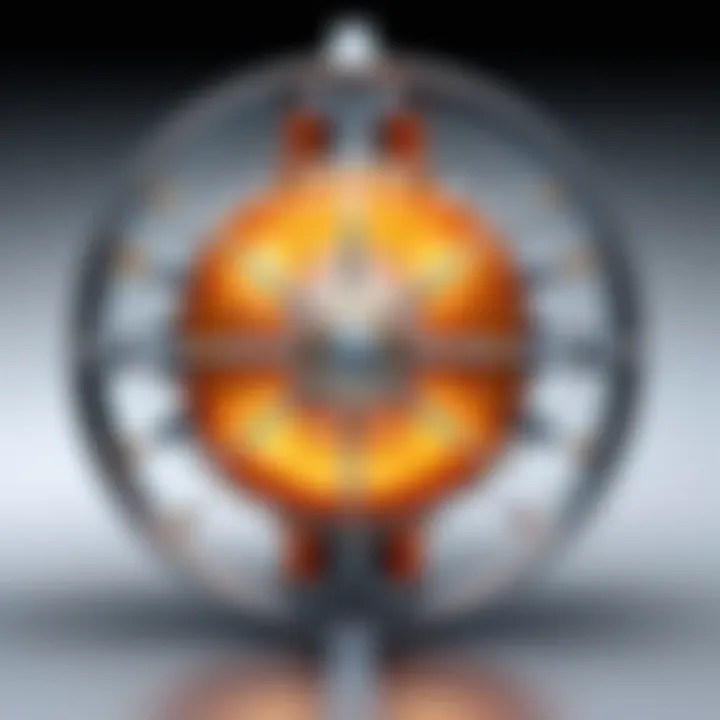
Intro
The landscape of energy production is on the brink of a transformative shift, and at the forefront lies a monumental technological endeavor—nuclear fusion reactors. Unlike their fission counterparts, which split atomic nuclei to release energy, fusion reactors strive to combine smaller atomic nuclei, akin to how the sun generates power. This process promises vast amounts of energy with minimal environmental impact. However, achieving practical fusion energy has been a long-standing challenge, fraught with complexities and hurdles.
In this comprehensive exploration, we will delve into the intricate mechanics of nuclear fusion, dissect the technological innovations driving these reactors, and consider the ramifications for future energy security. We’ll also highlight prominent figures and organizations spearheading this quest, examine safety parameters, and ponder the broader implications of fusion technology for global energy dynamics.
As we navigate through various dimensions of fusion reactors, the aim is to equip readers with a rich understanding of both the potential and the challenges they present. So buckle up, as we embark on a journey through the world of nuclear fusion.
Preface to Nuclear Fusion
The topic of nuclear fusion isn’t just a scientific curiosity; it has the potential to be a cornerstone of future energy production. Understanding nuclear fusion reflects an intersection of physics, engineering, and environmental consciousness. It represents a new frontier in energy technology which, if mastered, could provide vast amounts of clean energy, transforming our global energy landscape. This article aims to shed light on these significant aspects, examining the nuts and bolts of how these reactors operate and what they promise for sustainable energy.
Definition and Basics of Nuclear Fusion
Nuclear fusion is the process where two light atomic nuclei combine to form a heavier nucleus, releasing considerable energy in the process. Think of it as the cosmic equivalent of a heavyweight boxing match, but instead of punches, it’s hydrogen atoms colliding at extreme temperatures and pressures. For instance, the sun generates energy through fusion by converting hydrogen into helium. This is not just a starry concept — it’s a fundamental reaction happening all around us.
In practical terms, for a fusion reaction to occur, specific conditions must be met: extreme heat and density. These conditions allow the positively charged nuclei to overcome their mutual repulsion and collide. The energy produced from this reaction can theoretically power cities and industries while producing minimal radioactive waste. Contrast this with current fission reactors, where Uranium atoms are split apart, resulting in more long-lived radioactive waste.
Moreover, fusion fuels, such as deuterium and tritium, are abundant. For instance, deuterium can be extracted from seawater, presenting a critical advantage in sustainability. As we explore further in this article, understanding these basic elements of fusion is vital in grasping its full implications on our energy future.
Historical Context of Fusion Research
The journey into nuclear fusion research has been long and intricate. From the early 20th century when scientists such as Albert Einstein and Niels Bohr laid down the rudimentary principles of nuclear physics, the dream of harnessing fusion has intrigued many. In the 1940s and 1950s, the onset of the Cold War led to an intensified race for energy solutions, prompting research into both fission and fusion.
One key benchmark in fusion research was the development of the Tokamak model by Soviet physicists in the 1950s. The Tokamak design aimed to confine the hot plasma necessary for fusion using magnetic fields. This design remains one of the most researched configurations to this day. While progress was steady, achieving self-sustaining fusion has remained elusive, often likened to catching lightning in a bottle.
By the late 20th and early 21st centuries, global initiatives gathered momentum. The ITER project, an international effort in France, symbolizes a significant leap forward in fusion research. The collaboration among nations aims to demonstrate the feasibility of fusion as a large-scale energy source.
However, challenges remain — political, technological, and societal hurdles complicate the path to achieving functional fusion energy. As we probe deeper into the intricacies of fusion reactors, it becomes apparent that both a historical perspective and an understanding of current initiatives are essential for any discussion about the future of nuclear fusion.
Fundamentals of Fusion Reactions
Understanding the fundamentals of fusion reactions is crucial for anyone interested in the future of energy production. These reactions hold the potential to revolutionize how we harness power, providing a nearly limitless source of energy while minimizing harmful emissions. As the world grapples with the challenges posed by climate change and dwindling fossil fuels, fusion offers a beacon of hope. Exploring these fundamentals not only unpacks the science behind fusion but also highlights the exciting possibilities that arise from its successful implementation.
The Fusion Process Explained
Fusion is fundamentally the process by which two light atomic nuclei combine to form a heavier nucleus, releasing an enormous amount of energy in the process. The most commonly cited example involves isotopes of hydrogen—deuterium and tritium. When these isotopes collide and fuse, they form helium and release a neutron along with an impressive amount of energy. The equation representing this reaction is:
[ ^2_1H + 3_1H \rightarrow 4_2He + 1_0n + Energy ]
In practice, achieving the conditions necessary for fusion is nothing short of a scientific feat. It requires extremely high temperatures, reaching up to 15 million degrees Celsius, as found in the sun. At these temperatures, the kinetic energy of the nuclei is sufficient to overcome their mutual electrostatic repulsion—a barrier that is often dubbed as the Coulomb barrier. To maintain such temperatures and create the necessary pressure, innovative reactor designs like Tokamaks and Stellarators are developed.
"The core of every star is a fusion reactor, showcasing the power of combining hydrogen atoms into helium, an energy source that has powered the universe for billions of years."
Fusion’s cleanliness is striking; it produces minimal radioactive waste compared to fission reactors, making it an appealing option for energy generation. Understanding this process lays the groundwork for examining the various designs and advancements that can facilitate practical fusion energy.
Types of Fusion Reactions
Fusion reactions can be categorized based on the materials used and the conditions in which they are conducted. Here are the primary types:
- Deuterium-Tritium (D-T) Fusion: This is the most researched fusion reaction. It relies on combining deuterium and tritium. The energy yield is exceptionally high, making it a prime candidate for future reactors.
- Deuterium-Deuterium (D-D) Fusion: In this reaction, two deuterium nuclei may collide. While the energy output is lower than D-T fusion, it does enable the possibility of using ordinary seawater as fuel, given that deuterium is abundantly available in it.
- Proton-Proton (p-p) Chain: Predominantly occurring in stars the size of our sun, this chain reaction is a series of steps resulting in the fusion of hydrogen into helium. The process is slow and complex, often taking millions of years.
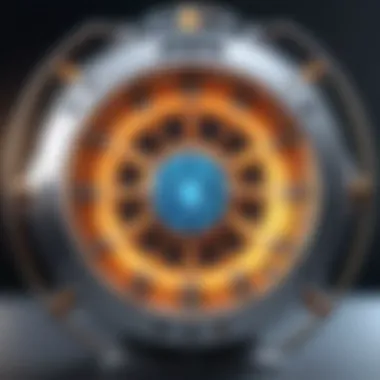
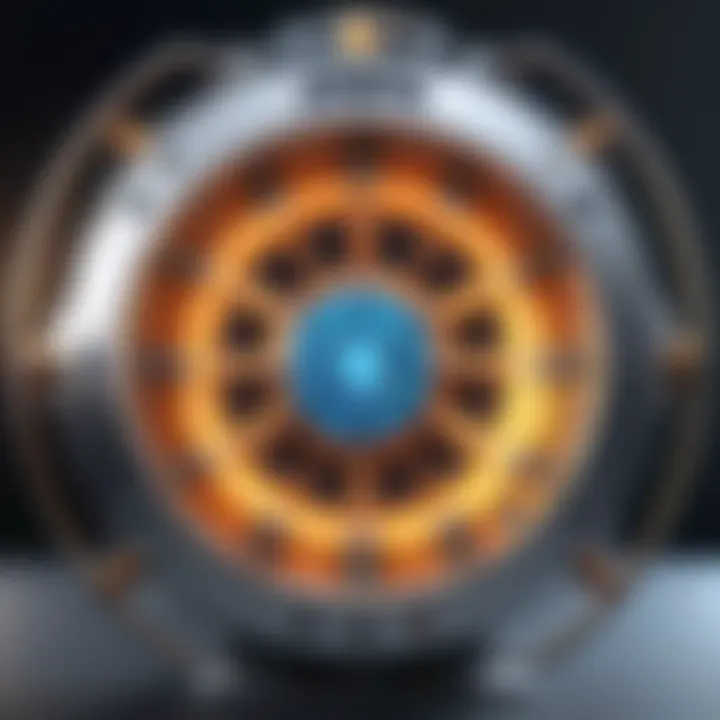
The fusion process essentially revolves around generating immense amounts of energy through these reactions, setting the stage for what is hoped to be a viable alternative to current energy systems. Each type of fusion reaction presents unique advantages and challenges, all of which contribute to the broader landscape of fusion energy research.
Design and Components of Fusion Reactors
The architecture of fusion reactors is no small potatoes. The design isn’t just about looking sleek or futuristic; it’s the backbone of how effectively energy can be produced. Various reactor types come with their own sets of advantages, challenges, and applications. Understanding how these reactors are built raises questions of efficiency and safety that can make or break future energy solutions. This section will dive into three prominent reactor designs: the Tokamak, Stellarator, and Inertial Confinement Fusion systems, inherently focusing on the core elements that define their structure and purpose.
Tokamak: The Most Common Reactor Design
The Tokamak design has gained considerable traction since its inception in the 1950s. The name means "toroidal chamber with magnetic coils," and this design relies heavily on magnetic fields to confine hot plasma. Crucially, this method is effective at reducing the possibility of energy loss due to contact with reactor walls. Copper and superconducting coils create powerful magnetic fields, ensuring the plasma remains stable.
However, this design isn’t without its flaws. The demand for high magnetic field strengths can lead to significant energy consumption. Still, the Tokamak is heralded for its progress in enabling long-duration plasma confinement. It serves as a key player in current fusion research efforts, such as the ITER project in France, which aims to demonstrate the feasibility of large-scale fusion energy.
Stellarator: An Alternative Design
On the flip side, the Stellarator offers an alternative perspective. Unlike the Tokamak, it doesn’t rely on a current induced in the plasma for stability. Instead, it employs twisted magnetic fields, which allow for a more steady plasma state without the same power draw. This clever twist might sound like a great idea in theory, but taking it from the drawing board to real-world applications presents its own challenges.
Stellarators are often complex to design and fabricate, often leading to difficulties with consistency in plasma stability. The variation in magnetic configuration can produce unpredictable results. Nevertheless, facilities like Wendelstein 7-X in Germany are pushing the envelope, attempting to overcome these hurdles.
In a world where energy efficiency is paramount, Stellarators might one day offer a viable method for achieving sustainable fusion energy without the constant power needs of the Tokamak.
Inertial Confinement Fusion
Steering away from magnetic confinement, Inertial Confinement Fusion (ICF) targets plasma by compressing it rapidly using lasers or other energy sources. The aim here is to generate conditions akin to those found in the core of stars, thus achieving fusion. This method relies on precise timing and the influx of energy in a split second to monitor how fuel pellets react.
The National Ignition Facility in the United States stands as a testament to ICF’s potential. It houses LANL’s experiments that utilize enormous laser systems to compress and heat tiny fuel pellets. Fascinatingly, while ICF can achieve high temperatures, the balance of creating self-sustaining reactions remains a tricky business. Achieving a significant return on energy investment has been elusive but is under constant scrutiny by researchers worldwide as a potential game-changer for fusion energy.
In summary, the design of fusion reactors plays a pivotal role in their functioning and potential to produce energy sustainably. Each method, while unique, presents its own set of challenges that need to be comprehended to move forward effectively.
Technological Advances in Fusion Energy
The realm of nuclear fusion is not standing still; it's a fast-paced arena filled with developments that promise to transform energy production as we know it. These advances hold the potential not just to enhance efficiency but also to make fusion reactors more viable alternatives to conventional energy sources like fossil fuels.
Fusion energy stands at a critical juncture, pushing the boundaries of what humanity can achieve in energy technology. With advances coming in leaps and bounds, understanding fuson innovations helps to grasp how they could practically influence energy systems and sustainability on a grand scale.
Recent Breakthroughs and Innovations
In recent years, several significant breakthroughs in fusion technology have captured the imagination of scientists and investors alike. One prime example is the development of high-temperature superconductors. These materials, which can conduct electricity without resistance at relatively high temperatures, allow for more efficient magnetic confinement in fusion reactors. The implication is profound: with better superconductive magnets, fusion reactors can reach the necessary plasma temperatures more quickly and efficiently.
Another noteworthy advancement is the use of advanced computational techniques, such as artificial intelligence and machine learning. Researchers harness these technologies to optimize reactor designs and predict plasma behavior. This data-driven approach can essentially foresee reactor performance under varying conditions, thus enhancing stability and efficiency in ongoing fusion experiments.
"The innovations happening in fusion research are not just academic; they are significant steps toward a viable fusion energy future."
Furthermore, facilities like the National Ignition Facility (NIF) in the United States have achieved pivotal milestones in inertial confinement fusion. In 2021, NIF reported a breakthrough in energy gain during their fusion experiments, which has bolstered hopes that fusion could one day become a reliable energy source. Each of these breakthroughs feeds into a larger narrative of progress, steering us toward a cleaner, brighter energy future.
Future Prospects and Experimental Technologies
Looking forward, the landscape of fusion energy teems with possibilities. One of the most exciting prospects is the development of fusion power plants that could be operational by the 2030s. Such plants are expected to balance the grid alongside renewable sources. Companies like Helion Energy and institutions like the International Thermonuclear Experimental Reactor (ITER) are spearheading efforts to create operational reactors that could deliver energy directly to homes and businesses.
Additionally, hybrid fusion-fission reactors show great promise. These systems combine fusion processes with traditional fission reactors, essentially using the neutrons produced in fusion reactions to trigger fission in a sustainable way. This hybrid approach aims to effectively generate more energy while utilizing the existing capabilities of fission technology.
Among the many experimental technologies being explored, plasma-facing materials are crucial. Materials that can withstand extreme temperatures and neutron bombardment are necessary for the longevity of future reactors. Innovations in these areas focus on reducing reactor wear and extending operational lifespans, a vital component for economic viability.
In summary, the advances in fusion technology represent not just a race toward better energy solutions but also a reflection of human ingenuity. As we continue to learn from failures and successes alike, the hope for fusion energy remains brighter than ever, poised to change the very fabric of energy supply.
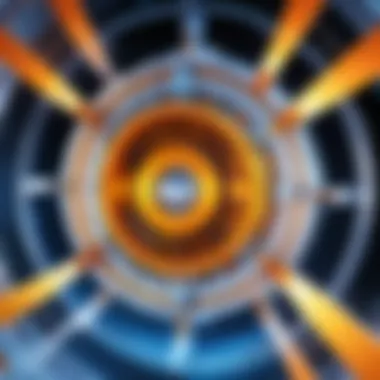
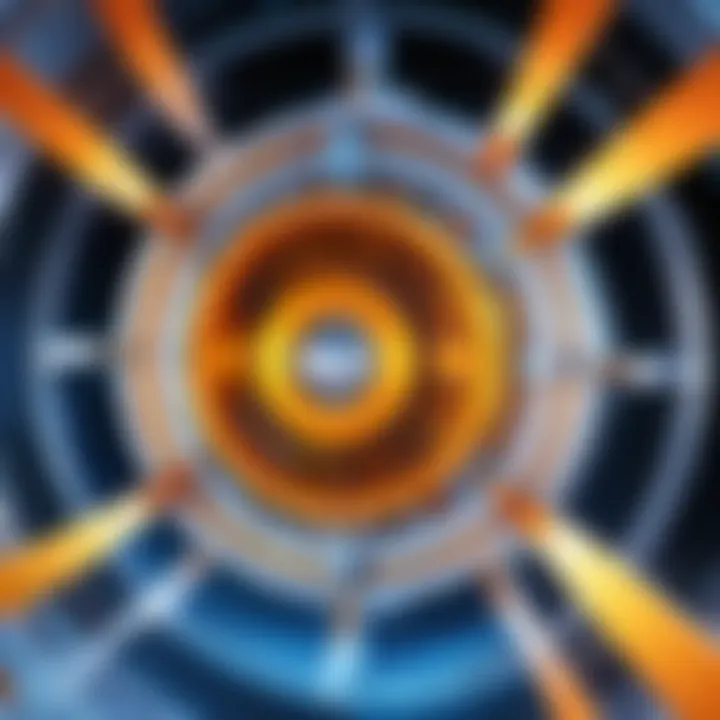
Safety Considerations and Challenges
Nuclear fusion, while holding the potential for substantial energy production, brings a set of safety considerations that must be thoroughly addressed. Understanding these challenges is key to the broader acceptance and implementation of fusion technology. A nuclear fusion reactor must mitigate risks not only to the environment but also to human health, as these factors play a major role in the overall perception and viability of fusion as a safe energy source.
Radiation and Environmental Safety
When one thinks of nuclear energy, radiation often comes to mind. In the context of fusion, however, the picture is quite different from that of fission reactors. Fusion reactions can produce neutron radiation, which is not negligible. This radiation can activate materials in the reactor, leading to long-term waste management challenges. Furthermore, with neutrons being released, it's essential to maintain shielding to protect the working environment and surrounding areas.
- Active Materials: Neutron activation differs from fission's radioactive waste. Some materials can become hazardous once they have been exposed to neutron bombardment. This means selecting materials for reactor construction is crucial. For instance, lithium is often chosen due to its relatively lower activation when exposed to neutron radiation.
- Environmental Impact: The need to assess long-term impacts on ecosystems cannot be overlooked. Strategies for waste disposal and environmental monitoring must be clearly established to provide reassurance of safety not only during operation but also post-operation.
Overall, understanding and addressing radiation risks—both in terms of reactor safety and environmental integrity—is essential for demonstrating nuclear fusion as a clean energy alternative.
Technical Challenges in Engineering and Operation
Engineering and operational challenges pose significant hurdles to the successful deployment of nuclear fusion reactors. Achieving and maintaining the conditions necessary for hydrogen isotopes to collide and fuse requires an intricate balance of technology and ingenuity. Here are some of the notable challenges in this domain:
- Plasma Stability: Controlling a plasma at such high temperatures (over 100 million degrees Celsius) is no small feat. Achieving stability is often compared to trying to hold jello in your hands; it’s temperamental and easily disrupted. This leads to issues like edge plasma instability, which can affect the efficiency and longevity of a reactor.
- Material Durability: The materials used within fusion reactors face extreme conditions. Not only do they need to endure high temperatures, but they also must withstand bombardment from fast neutrons. New alloys and composite materials are under research to see how they perform over time in these conditions.
- Engineering Complexity: The design of the reactor itself is no walk in the park. The intricate setup of magnetic confinement, fuel injection systems, and cooling mechanisms requires precision engineering. For example, the tokamak design emphasizes the necessity of an advanced understanding of electromagnetic principles to maintain confinement.
- Operational Costs: Despite its potential, the financial viability cannot be ignored. Developing fusion technology demands significant upfront investments, not to mention ongoing operational costs. Companies and governments must balance this with the expected return on investment in a world eager for sustainable energy sources.
In summary, tackling the technical challenges involved in the engineering and operation of fusion reactors is critical. Only through innovation and dedication can the dream of a sustainable fusion energy future be realized.
Economics of Nuclear Fusion Energy
The realm of nuclear fusion energy is not just a scientific utopia but a potential economic powerhouse waiting to be tapped. Understanding the financial aspects involved is crucial for gauging how fusion could revolutionize energy production while remaining competitive in a landscape dominated by fossil fuels and renewables. It’s not just about the shiny tech; it’s about economics, policy implications, and market dynamics that will shape its future viability.
Cost Analysis of Fusion Projects
When we dive into the cost analysis of fusion projects, a few major players stand out. Developing fusion reactors requires enormous upfront investments that can easily run into billions. The construction of facilities such as ITER (International Thermonuclear Experimental Reactor) showcases this. The ITER project, ongoing in France, has an estimated cost upwards of €20 billion.
Here are a few key points to consider in this analysis:
- Initial Investment: Most of the expenses are tied to research and construction. Unlike wind or solar power, which can be scaled in smaller projects, fusion requires a significant initial outlay.
- Operating Costs: Fusion plants could be more efficient over time, leading to lower running costs compared to current fission plants.
- Technological Innovation: Every advance can potentially lower costs. Factors such as improved materials and efficiencies in the designs of reactors play a crucial role.
- Government Funding: Many fusion projects receive substantial backing from governments (think of the trillions spent on the initial atomic energy ventures).
“The true cost of fusion will only be realized as projects transition from theory to practice.”
Understanding these elements provides clarity about why fusion has not yet made its commercial debut despite its promise. Balancing initial costs with long-term benefits is part of the ongoing conversation.
Potential for Energy Market Impact
The implications of nuclear fusion extend far beyond the lab environment. If fusion energy can be successfully harnessed on a commercial scale, it has the potential to reshape the global energy marketplace drastically. Let’s explore some of these impacts:
- Energy Security: Providing a virtually limitless energy source could reduce reliance on imported fuels. Countries wouldn’t have to worry about volatile oil prices and can focus on sustainable energy.
- Job Creation: As new industries around fusion technology emerge, job opportunities in engineering, maintenance, and project management could multiply.
- Price Stabilization: The introduction of a stable, low-cost energy source can create ripple effects across various sectors—leading to lower prices for consumers and businesses.
- Environmental Benefits: Given the minimal radioactive waste and reduced carbon emissions, fusion stands to gain traction in an increasingly climate-conscious market.
The fusion energy market is still in its nascent stages, but the transformative potential is undeniable. Markets already fluctuate based on energy trends; adding fusion could create new dynamics and opportunities that we can only begin to imagine as the technology matures.
Key Players in the Fusion Landscape
Understanding the key players in the nuclear fusion landscape is crucial for grasping how advancements in this field will shape our future energy environment. A variety of stakeholders, ranging from government entities to private corporations and international research organizations, play a significant role in fusion research and development. Their collective efforts contribute not only to technological innovations but also influence public perception and policy related to fusion energy.
Government Initiatives and Funding
Governments around the globe have recognized the transformative potential of nuclear fusion and are committing resources to explore it. For instance, the United States Department of Energy has recently earmarked substantial funding for projects aimed at accelerating fusion research. This financial support can be pivotal in bringing theoretical concepts closer to reality.
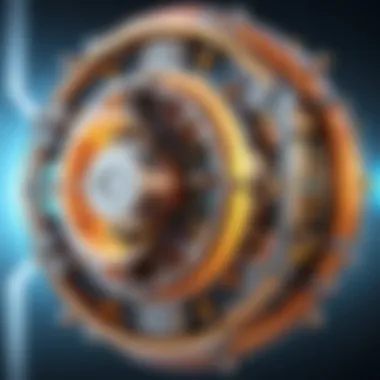
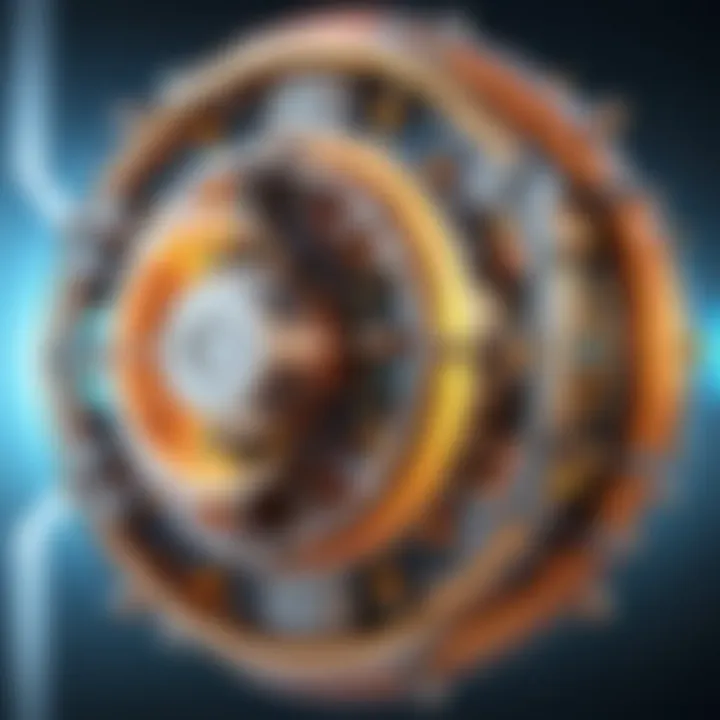
Various countries are investing in fusion through national laboratories and academic institutions. In the United Kingdom, the ITER project symbolizes international cooperation and ambition, with nations pooling resources to develop a nuclear fusion reactor. Such investment not only advances scientific knowledge but also showcases a commitment to sustainable energy solutions. This collaboration among states leads to a pooling of expertise, minimizing duplication of efforts and maximizing the impact of research.
Private Sector Involvement in Fusion Development
The private sector is becoming increasingly active in the fusion domain, where companies like Helion Energy and TAE Technologies are making waves against the backdrop of traditional research institutions. These firms often operate with different funding models compared to government projects, sometimes relying on private investments and venture capital. This encourages innovation and agility, allowing them to explore distinct approaches that might not be feasible within governmental constraints.
For example, Helion Energy aims for a more compact and economically viable fusion reactor, promising to reduce energy costs while also addressing climate change. Such endeavors from private companies are essential not only for technological advancement but also because they foster competition, which can lead to quicker breakthroughs.
International Collaborations and Research
Fusion research does not happen in isolation. The global nature of the issue has sparked numerous collaborative efforts among countries. Projects like ITER in France represent a significant global partnership involving multiple nations, such as the EU, USA, Chinese, Russia, India, and Japan. The intricacies of such partnerships include sharing knowledge, technology, and resources, which can lead to faster progress than isolated efforts.
Collaboration spans beyond projects as countries share research findings and best practices. For instance, many fusion scientists attend international conferences, where they exchange insights and learn from one another about their latest findings and challenges. Collaborative networks, forums, and platforms facilitate the sharing of data that might otherwise be locked within national interests.
"Transformative progress in nuclear fusion is often driven by collaborative efforts that transcend borders, emphasizing the importance of shared knowledge in addressing global energy challenges."
The interplay between public institutions, private companies, and international partnerships shapes the future of fusion energy, demonstrating that the path to viable nuclear fusion lies in a concerted effort across various sectors.
Ethical and Societal Implications
As we step into the future of energy solutions, the ethical and societal implications of nuclear fusion reactors demand serious attention. This isn't just a matter of technology; it's about balancing innovation with responsibility. The consideration of fusion technology presents an opportunity to rethink our relationship with energy, while addressing ethical questions that accompany any transformative discovery.
Public Perception of Nuclear Fusion
Public perception of nuclear fusion is, understandably, a mixed bag. Many individuals view nuclear energy through the lens of fear, shaped largely by the catastrophic failures of fission reactors in the past. Incidents like Chernobyl and Fukushima loom large in public memory, overshadowing the potential benefits of fusion. This presents a significant hurdle for advocates wanting to introduce fusion as a viable energy source.
People tend to associate nuclear technology with danger, which can lead to skepticism and resistance. However, fusion differs notably from fission in its risk profile. The fundamental mechanics of fusion make it inherently safer. When fusion reactions occur, they do not produce long-lived radioactive waste, which is often a bone of contention in the nuclear discourse. Moreover, the fuels used, like hydrogen isotopes, are more abundant and less harmful.
A 2019 survey indicated that about 54% of the public would support research into fusion technology if it could lead to cleaner energy. This light at the end of the tunnel illustrates a growing curiosity amongst people about how fusion could serve as a solution to climate change and energy scarcity. Engaging the public through education and transparent dialogue can shift perceptions toward a more favorable view of fusion.
"Public discourse plays a pivotal role in shaping the potential acceptance of fusion energy. Without addressing fears, progress risks stagnation."
Addressing Ethical Concerns in Fusion Research
As with any advanced technology, ethical concerns are rife in discussions on fusion research. One pressing issue revolves around the potential military applications of fusion technology. The same science that could provide unlimited energy might also lead to powerful weapons if misappropriated. Thus, regulatory frameworks need to be established early on to prevent the misuse of fusion capabilities. This will involve collaboration among governments, scientists, and ethicists to create guidelines that ensure responsible use of fusion technologies.
Next, equity in access to fusion energy must be a focal point. Should fusion become a reality, it’s vital that its benefits be shared equitably across societies, rather than becoming trapped in wealthy infrastructures. This brings to light the challenge of energy justice, which advocates for equal access to resources regardless of socioeconomic status. Building a framework that ensures these technologies reach those underserved by current energy grids can drastically affect energy poverty on a global scale.
Lastly, there’s the environmental angle. While fusion is much cleaner than fossil fuels, concerns still exist regarding its impact on our ecosystems. Researchers must heed the ecological footprints of fusion reactors and the mining of raw materials needed for reactor construction. Sustainable practices need to be woven into the very fabric of fusion energy production, reducing harm to our planet.
Culmination
In reflecting on the realms of nuclear fusion reactors, it becomes clear that the topic is not just an academic exercise but rather a gateway to reshaping our energy landscape. The synthesis of numerous factors underscores the importance of comprehending the nuances involved.
Summary of Current State and Future Directions
Current advancements in fusion technology are akin to the first strides in aviation—every little success paves the way for a monumental leap. Today, designs like the Tokamak and Stellarator are being fine-tuned continually, aiming at operational efficiency and prolonged stability. The journey isn't linear; it features many stops, stumbles, and spectacular triumphs. Key players in both the public and private sectors are pouring resources into fusion projects that exhibit promising results. For instance, projects like ITER in France are international pursuits that promise not only innovation but also collaboration across borders.
The future, however, demands continued vigilance regarding funding and research priorities. Areas like radiation safety and environmental implications require unceasing scrutiny. As technology progresses, so must our ethical considerations; balancing our hunger for energy with responsibilities to the planet and to future generations is paramount.
Final Thoughts on the Role of Fusion Energy
Fusion energy, often described as the "holy grail" of energy sources, holds immense promise. Theoretically, it's abundant and cleaner than traditional methods of energy production. The excitement comes from the potential to harness the very forces that power the stars. Yet, as we step into a future where this energy source could blossom, cautionary tales of past energy projects remind us that optimism must also come with a measure of skepticism.
Achieving sustainable fusion energy isn't merely a scientific challenge; it's a societal endeavor that intertwines tech, ethics, and global collaboration. The faster we can shift towards harnessing this power, with an eye on safety and equity, the more tangible the dream of a sustainable energy future will become.
"The world is as it is, and our energy future must reflect the ambition of the best we have to offer."
Fusion reactors have the capacity to transform global energy dynamics—if we tread wisely.